Beringian wolf

The Beringian wolf was a hypercarnivorous ecomorph of the gray wolf that inhabited eastern Beringia (modern day Alaska) and northern Wyoming during the Late Pleistocene and early Holocene. It was similar in size to the modern Alaska wolf and other Pleistocene wolves but was more robust with a shorter, broader palate and large carnassial teeth relative to its overall skull size. This adaptation allowed it to produce relatively large bite forces, grapple with large struggling prey, and therefore to predate and scavenge on Pleistocene megafauna and in particular horse and bison.[1][2]
The close of the Ice Age led to the Quaternary extinction event with its loss of mainly large animals that are referred to as Pleistocene megafauna. It is proposed that with the loss of this prey the Beringian wolf also went extinct. Another proposal is that they were out-competed by an invasion of the ancestor of today's gray wolf.
Taxonomy and evolution
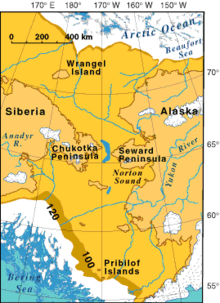
In 1975, a study was undertaken of canid remains dated to the Late Pleistocene and early Holocene that had been uncovered decades earlier by miners around Fairbanks, Alaska and described as "short-faced wolves". The study classified these as Canis lupus.[3] Later genetic analyses supported this classification,[1][4][5] with the name eastern Beringian wolf being designated to the specimens. Beringia was an area of land that once spanned the Bering sea from Eurasia to North America in the time these wolves existed.
A study on the skeletal material from 56 eastern-Beringian wolves based on radio carbon dating showed a continuous population from over 50,800 years before present (YBP)[6] to 12,500 YBP, and one single wolf dated at 7,600 YBP. This indicates that their population was in decline after 12,500 BP,[1] although megafauna was still available in this region until 10,500 BP. The timing of this latter specimen is supported by the recovery of mammoth and horse DNA from sediments dated 10,500 YBP - 7,600 YBP from the interior of Alaska.[7]
Basal wolf
One study found the Beringian wolf to be basal to all other gray wolves except for the extant Indian gray wolf and the extant Himalayan wolf.[1] Another two studies found the Beringian wolf to be basal to all other wolves except for an extinct wolf from the Medvezya cave, Russia and the extinct Belgium clade (the Himalayan wolf and the Indian gray wolf were not included in these two studies).[5][8]
Two types of wolf
A haplotype (haploid genotype) is a group of genes in an organism that are inherited together from a single parent.[9][10] A haplogroup is a group of similar haplotypes that share a common ancestor with a single-nucleotide polymorphism mutation.[11][12] Mitochondrial DNA passes along the maternal lineage that can date back thousands of years.[11]
In 2010, a study compared a 230 base pair sequence of the mitochondrial control region from 24 ancient wolf specimens from western Europe dated between 44,000–1,200 YBP with those of modern gray wolves. Most of the sequences could be represented on a phylogenetic tree. The haplotypes of the Himalayan wolf and the Indian gray wolf could not because they were 8 mutations apart from the other wolves,[4] indicating distinct lineages which had previously been found in other studies.[4][13][14] The study found that there were 75 different gray wolf mDNA haplotypes that include 23 in Europe, 30 in Asia, 18 in North America, 3 in both Europe and Asia, and 1 in both Europe and North America.[4] These haplotypes formed two haplogroups that were separated from each other by 5 mutations. Haplogroup 1 formed a monophyletic clade (indicating that they all carried the same mutation inherited from a single female ancestor). All other haplotypes were basal in the tree, and these formed 2–3 smaller clades that were assigned to haplogroup 2 that was not monophyletic.[4][15]
Haplogroups 1 and 2 could be found spread across Eurasia but only haplogroup 1 could be found in North America. The ancient wolf samples from western Europe differed from modern wolves by 1 to 10 mutations, and all belonged to haplogroup 2 indicating a haplogroup 2 predominance in this region for over 40,000 years before and after the Last Glacial Maximum. A comparison of current and past frequencies indicated that in Europe haplogroup 2 became outnumbered by haplogroup 1 over the past several thousand years but in North America haplogroup 2 - the Beringian wolf - became extinct and was replaced by haplogroup 1 after the Last Glacial Maximum.[4][15] Access into North America was available between 20,000–11,000 YBO, after the Wisconsin glaciation had retreated but before the Bering land bridge became inundated by the sea.[16] Therefore, haplogroup 1 was able to enter into North America during this period. The specialized Pleistocene wolves did not contribute to the genetic diversity of modern wolves, and the modern wolf populations across the Holarctic are likely to be the descendants of wolves from populations that came from more southern refuges.[17]
A scenario consistent with the phylogenetic, ice sheet and sea-level data was that during the Ice Age when sea levels were at their lowest, there was a single wave of wolf colonization into North America starting with the opening of the Bering land bridge 70,000 YBP and closing during the Late Glacial Maximum of the Yukon corridor that ran through the division between the Laurentide Ice Sheet and the Cordilleran Ice Sheet 23,000 YBP. As wolves had been in the fossil record of North America but modern wolves could trace their ancestry back only 80,000 years, the wolf haplotypes that were already in North America were replaced by these invaders, either through competitive displacement or through admixture.[8] The replacement in North America of a basal population of wolves by a more recent one supported the findings of earlier studies.[4][15][1][8] There possibly existed a panmictic wolf population with gene flow spanning Eurasia and North America until the closing of the ice sheets.[4][17][8] Once the sheets closed, the southern wolves were isolated and north of the sheets only the Beringian wolf existed. The land bridge became inundated by the sea 10,000 YBP, the sheets receded 12,000–6,000 YBP, the Beringian wolf went extinct and the southern wolves expanded to recolonize the rest of North America. All North American wolves are descended from those that were once isolated south of the ice sheets. However, much of their diversity was later lost during the twentieth century.[8]
- For more details on this topic, see: Two wolf haplogroups
Dissenting view
In 2016, a study compared the mitochondrial DNA sequences of ancient wolf specimens and modern wolves, including specimens from the remote regions of North America, Russia and China. One ancient haplotype that had once existed in Alaska (Eastern Beringia 28,000 YBP) and Russia (Medvezya Cave 18,000 YBP) was shared with some modern wolves found living in China and Mongolia. A previous study had identified two wolf haplogroups,[4] however these were not clearly delineated in this study but it concurred that the genetic diversity of past wolves has been lost at the beginning of the Holocene in Alaska, Siberia, and Europe with limited overlap with modern wolves. For the ancient wolves of North America, instead of an extinction/replacement model suggested by a previous study,[1] this study found substantial evidence of a population bottleneck in North America in which the ancient wolf diversity was almost lost around the beginning of the Holocene (no further elaboration in the study). In Eurasia, the loss of ancient lineages could not be simply explained and appears to have been slow across time with the reasons unclear.[6]
Ecomorph adaption

Ecological factors including habitat type, climate, prey specialization and predatory competition will greatly influence gray wolf genetic population structure and cranio-dental plasticity.[18][19][20][21][22][23][24][25][26] Therefore, within the Pleistocene gray wolf population the variations between local environments would have encouraged a range of wolf ecotypes that were genetically, morphologically and ecologically distinct from one another.[26] The Beringian wolf demonstrates evolutionary cranio-dental plasticity not previously seen in North American Canis lupus.[1]
Paleoecology
The last glacial period, commonly referred to as the 'Ice Age', spanned 125,000 YBP[27] to 14,500 YBP[28] and was the most recent glacial period within the current ice age which occurred during the last years of the Pleistocene era.[27] The Ice Age reached its peak during the Last Glacial Maximum, when ice sheets commenced advancing from 33,000 YBP and reached their maximum positions 26,500 YBP. Deglaciation commenced in the Northern Hemisphere approximately 19,000 YBP and in Antarctica approximately 14,500 years YBP, which is consistent with evidence that this was the primary source for an abrupt rise in the sea level 14,500 YBP.[28] A vast, cold and dry Mammoth steppe stretched from Spain across Eurasia and over the Bering land bridge into Alaska and the Yukon where it was stopped by the Wisconsin glaciation. This land bridge existed because more of the planet's water was locked up in glaciation than now and therefore the sea-levels were lower. When the sea levels began to rise this bridge was inundated around 11,000 YBP.[29] The fossil evidence from many continents points to the extinction mainly of large animals, termed Pleistocene megafauna, near the end of the last glaciation. Beringia was a refuge for animals and plants because it received more moisture than the rest of the Mammoth steppe.
- See further: Beringian refugium
Diet
Stable isotope analysis conducted on the bone of a specimen allows researchers to form conclusions about the diet, and therefore the ecology, of extinct wolf populations. This analysis suggests that the Pleistocene wolves from haplogroup 2 found in both Beringia and in Belgium preyed mainly on Pleistocene megafauna,[4][1][30] which became rare at the beginning of the Holocene 12,000 years ago.[4][24] The Beringian wolf ate horse and bison, in addition to woodland muskox pre-galacial (50,000 YBP - 23,000 YBP) and mammoth after this time. This analysis supports the conclusion that they were capable of killing and dismembering large prey.[1] A more in-depth analysis of the fossils of a number of carnivores from the Fairbanks region of Alaska found that mammoth was rare in the diets of Beringian carnivores – Beringian wolves, lions, short-faced bears and the omnivorous brown bear. Half of the wolf specimens were found to be muskox and caribou specialists, and the other half were horse and bison specialists or generalists. Two full-glacial (23,000–18,000 YBP) wolves were found to be mammoth specialists but we cannot tell if this was due to scavenging or predation. The only survivor of this guild was the brown bear, an omnivore.[31]
Compared with extant gray wolves and Pleistocene gray wolves from Rancho La Brea, the eastern-Beringian ecomorph was hypercarnivorous, with a craniodental morphology more capable of capturing, dismembering, and consuming the bones of very large mega-herbivores, such as bison. When their prey disappeared, this wolf ecomorph did as well, resulting in a significant loss of phenotypic and genetic diversity within the species.[1] The timing of the extinction of horses in North America and the minimum population size for North American bison coincide with the extinction an entire wolf haplogroup in North America, supporting the notion that the disappearance of their prey caused the extinction of this wolf ecomorph.[24]
Skull and dentition

Beringian wolves were similar in physical size to the extant Alaskan wolf and the Pleistocene wolves found in Rancho La Brea (California) but were more robust with stronger jaws and teeth. Beringian wolves tended to have short, broad palates with large carnassials relative to their overall skull size. The row length of their premolars was longer, the P4 wider, and the M1 and M2 molars longer than other wolves. These features suggest a gray wolf adapted for producing relatively large bite forces. The short, broad rostrum increased the mechanical advantage of a bite made with the canine teeth and strengthened the skull against torsional stresses caused by struggling prey. Relatively deep jaws are characteristic of habitual bone crackers such as the spotted hyena, or canids that take prey as large or larger than themselves. Overall these features indicate that Beringian wolves were more specialized than modern gray wolves in killing and consuming relatively large prey and scavenging.[1][32]
In comparison to other gray wolf populations, Beringian wolf samples included many more individuals with moderately to heavily worn teeth. In addition, Beringian wolves exhibited heavier wear and significantly greater numbers of broken teeth. Overall fracture frequencies ranged from a low of 2% in Canis lupus irremotus (Canada, Idaho) to a high of 11% in the Beringian wolves. The distribution of fractures across the tooth row differs as well, with eastern-Beringian wolves having much higher fracture frequencies of incisors, carnassials, and molars. A similar pattern was observed in spotted hyenas, suggesting that increased incisor and carnassial fracture reflects habitual bone consumption, because bones are gnawed with incisors and subsequently cracked with the cheek teeth.[1]
In 2015, a study of the dental microwear on tooth enamel was undertaken for specimens of all of the carnivore species from Rancho La Brea, California, including remains of the large wolf Canis dirus guildayi (dire wolf) that was also a megafaunal hypercarnivore and exhibited a high incidence of tooth breakage. The evidence suggests that these carnivores were not food-stressed just before extinction and that carcass utilization was less than among large carnivores today. The high incidence of tooth breakage likely resulted from the acquisition and consumption of larger prey.[33]
Range
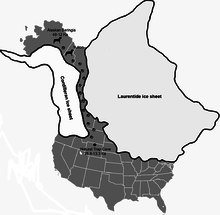
A similarly described robust ecomorph from the Late Pleistocene has been found in western Beringia (northeast Siberia)[35] but specimens have not been available for study.[1] The Late Pleistocene eastern-Beringian wolves have been found to be morphologically and genetically comparable to Late Pleistocene European wolves[36] and two studies found that some share a common haplotype,[4][1] which makes ecological similarity likely.[4] A study of the mDNA sequences from 20 eastern Beringian wolves yielded 16 haplotypes that were not found in modern wolves. However, the study found that a wolf from the Nerubajskoe-4 Paleolithic site, Ukraine dated 30,000 YBP, a wolf from the Zaskalnaya-9 Paleolithic site, Ukraine dated 28,000 YBP, and the "Altai dog" from the Altai Mountains of Central Asia dated 33,000 YBP had the same sequence as six Beringian wolves (indicating a common maternal ancestor). Another from the Vypustek cave, Czech Republic dated 44,000 YBP had the same sequence as two Beringian wolves (indicating another common maternal ancestor). Its genetic diversity was higher than that of its modern counterparts, implying that the wolf population of the Late Pleistocene was larger than at present.[1]
The Beringian wolves are phylogenetically associated with a distinct group of 4 modern European haplotypes (but these are not their direct descendents), which indicates that both ancient and extant North American wolves originated in Eurasia.[1] Two of these 4 haplotypes includes one only found in the Italian wolf and one only found in Rumania.[37] These 4 haplotypes fall with all of the Beringian wolf haplotypes under haplogroup 2[4] (see two types of wolf above). It has been proposed that the Pleistocene wolves across northern Eurasia and northern North America represented a continuous and almost panmictic population that was genetically and probably also ecologically distinct from the wolves living in this area today.[4][17]
Specimens that have been identified by morphology to be Beringian wolves and radiocarbon dated between 25,800-14,300 YBP have been found in the Natural Trap Cave at the base of the Bighorn Mountains in Wyoming, United States. The location is directly south of what would at that time have been the division between the Laurentide Ice Sheet and the Cordilleran Ice Sheet. A temporary channel between the glaciers may have existed from 25,800 YBP[34] until the advance of the ice sheets 16,000-13,000 YBP.[34][38] The migration of the Beringian wolf may have been the result of pursuing prey species, as the cave also contained specimens of Steppe bison that had migrated from Beringia and would have been prey for wolves,[34][39] and Muskox that is known to be an important prey species of the Beringian wolf.[34][31] Dire wolves were absent north of 42°N latitude in the Late Pleistocene, therefore this region would have been available for Beringian wolves to expand south along the glacier line. There is yet no evidence of expansion beyond this region.[34] Another study of bison through this area indicated that the corridor was closed between 23,000 YBP until 13,400 YBP,[40] suggesting that the wolves were unable to return north during this time.
Ancient specimens of megafaunally adapted wolves with similar skull and dentition have been found in western Beringia, the Taimyr Peninsula, the Ukraine, and in Germany where it is classified as C. l. spelaeus - the cave wolf.[35]
Competitors
The eastern-Beringian wolf was well positioned as the dominant large, pack-hunting canid within a predator guild that included the Saber-toothed cat, Beringian cave lion, bears (ursids), and two smaller canids, the dhole and coyote. The high frequency of tooth fracture indicates higher carcass consumption due to higher carnivore density and increased competition compared to modern wolves.[1] One study found that the Pleistocene coyote of 10,000 years ago was more carnivorous, much larger, and with skulls and jaws significantly thicker and deeper than those of recent populations.[41][42]
The large Late Pleistocene carnivores that were more carnivorous than their competitors faced greater vulnerability to extinction just prior to the Holocene. The Beringian cave lion, saber-toothed cat, and short-faced bear went extinct as did their large megafaunal prey, including the horse in North America. The coyote, black and brown bears, puma and bob-cat survived. Both the dire wolf and the Beringian wolf became extinct, leaving only the less carnivorous and more gracile form of the wolf to thrive.[1]
References
- 1 2 3 4 5 6 7 8 9 10 11 12 13 14 15 16 17 18 Leonard, J. A.; Vilà, C; Fox-Dobbs, K; Koch, P. L.; Wayne, R. K.; Van Valkenburgh, B (2007). "Megafaunal extinctions and the disappearance of a specialized wolf ectomorph" (PDF). Current Biology. 17 (13): 1146–50. doi:10.1016/j.cub.2007.05.072. PMID 17583509.
- ↑ Sam Turvey (2009). Holocene Extinctions. Oxford University Press. p. 257. ISBN 978-0-19-953509-5.
- ↑ Stanley J. Olsen (1985). Origins of the Domestic Dog: The Fossil Record – Chapter 2. University of Arizona Press.
- 1 2 3 4 5 6 7 8 9 10 11 12 13 14 15 Pilot, M. G.; Branicki, W.; Jędrzejewski, W. O.; Goszczyński, J.; Jędrzejewska, B. A.; Dykyy, I.; Shkvyrya, M.; Tsingarska, E. (2010). "Phylogeographic history of grey wolves in Europe". BMC Evolutionary Biology. 10: 104. doi:10.1186/1471-2148-10-104. PMC 2873414
. PMID 20409299.
- 1 2 Thalmann, O.; Shapiro, B.; Cui, P.; Schuenemann, V. J.; Sawyer, S. K.; Greenfield, D. L.; Germonpré, M. B.; Sablin, M. V.; López-Giráldez, F.; Domingo-Roura, X.; Napierala, H.; Uerpmann, H-P.; Loponte, D. M.; Acosta, A. A.; Giemsch, L.; Schmitz, R. W.; Worthington, B.; Buikstra, J. E.; Druzhkova, A.; Graphodatsky, A. S.; Ovodov, N. D.; Wahlberg, N.; Freedman, A. H.; Schweizer, R. M.; Koepfli, K.-.P.; Leonard, J. A.; Meyer, M.; Krause, J.; Pääbo, S.; Green, R. E.; Wayne, R. K. (2013). "Complete Mitochondrial Genomes of Ancient Canids Suggest a European Origin of Domestic Dogs". Science. 342 (6160): 871–74. Bibcode:2013Sci...342..871T. doi:10.1126/science.1243650. PMID 24233726.
- 1 2 Ersmark, Erik; Klütsch, Cornelya F. C.; Chan, Yvonne L.; Sinding, Mikkel-Holger S.; Fain, Steven R.; Illarionova, Natalia A.; Oskarsson, Mattias; Uhlén, Mathias; Zhang, Ya-Ping; Dalén, Love; Savolainen, Peter (2016). "From the Past to the Present: Wolf Phylogeography and Demographic History Based on the Mitochondrial Control Region". Frontiers in Ecology and Evolution. 4. doi:10.3389/fevo.2016.00134.
- ↑ Haile, J.; Froese, D. G.; MacPhee, R. D. E.; Roberts, R. G.; Arnold, L. J.; Reyes, A. V.; Rasmussen, M.; Nielsen, R.; Brook, B. W.; Robinson, S.; Demuro, M.; Gilbert, M. T. P.; Munch, K.; Austin, J. J.; Cooper, A.; Barnes, I.; Moller, P.; Willerslev, E. (2009). "Ancient DNA reveals late survival of mammoth and horse in interior Alaska". Proceedings of the National Academy of Sciences. 106 (52): 22352–22357. Bibcode:2009PNAS..10622352H. doi:10.1073/pnas.0912510106. PMC 2795395
. PMID 20018740.
- 1 2 3 4 5 Koblmüller, Stephan; Vilà, Carles; Lorente-Galdos, Belen; Dabad, Marc; Ramirez, Oscar; Marques-Bonet, Tomas; Wayne, Robert K.; Leonard, Jennifer A. (2016). "Whole mitochondrial genomes illuminate ancient intercontinental dispersals of grey wolves (Canis lupus)". Journal of Biogeography. doi:10.1111/jbi.12765.
- ↑ Cox, C. B.; Moore, Peter D.; Ladle, Richard (2016). Biogeography: An Ecological and Evolutionary Approach. Wiley-Blackwell. p. 106. ISBN 978-1-118-96858-1.
- ↑ Editorial Board (2012). Concise Dictionary of Science. New Delhi: V&S Publishers. p. 137. ISBN 978-93-81588-64-2.
- 1 2 Arora, Devender; Singh, Ajeet; Sharma, Vikrant; Bhaduria, Harvendra Singh; Patel, Ram Bahadur (2015). "Hgs Db: Haplogroups Database to understand migration and molecular risk assessment". Bioinformation. 11 (6): 272–5. doi:10.6026/97320630011272. PMC 4512000
. PMID 26229286.
- ↑ "Genetics Glossary". International Society of Genetic Genealogy. 2015. Retrieved 22 July 2016.
- ↑ Aggarwal, R. K.; Kivisild, T.; Ramadevi, J.; Singh, L. (2007). "Mitochondrial DNA coding region sequences support the phylogenetic distinction of two Indian wolf species". Journal of Zoological Systematics and Evolutionary Research. 45 (2): 163–172. doi:10.1111/j.1439-0469.2006.00400.x.
- ↑ Sharma, D. K.; Maldonado, J. E.; Jhala, Y. V.; Fleischer, R. C. (2004). "Ancient wolf lineages in India". Proceedings of the Royal Society B: Biological Sciences. 271 (Suppl 3): S1–S4. doi:10.1098/rsbl.2003.0071. PMC 1809981
. PMID 15101402.
- 1 2 3 Randi, Ettore (2011). "Genetics and conservation of wolves Canis lupus in Europe". Mammal Review. 41 (2): 99–111. doi:10.1111/j.1365-2907.2010.00176.x.
- ↑ Tamm, E.; Kivisild, T.; Reidla, M.; Metspalu, M.; Smith, D. G.; Mulligan, C. J.; Bravi, C. M.; Rickards, O.; Martinez-Labarga, C.; Khusnutdinova, E. K.; Fedorova, S. A.; Golubenko, M. V.; Stepanov, V. A.; Gubina, M. A.; Zhadanov, S. I.; Ossipova, L. P.; Damba, L.; Voevoda, M. I.; Dipierri, J. E.; Villems, R.; Malhi, R. S. (2007). Carter, Dee, ed. "Beringian Standstill and Spread of Native American Founders". PLoS ONE. 2 (9): e829. Bibcode:2007PLoSO...2..829T. doi:10.1371/journal.pone.0000829. PMC 1952074
. PMID 17786201.
- 1 2 3 Hofreiter, Michael (2007). "Pleistocene Extinctions: Haunting the Survivors". Current Biology. 17 (15): R609–11. doi:10.1016/j.cub.2007.06.031. PMID 17686436.
- ↑ Leonard, Jennifer (2014). "Ecology drives evolution in grey wolves" (PDF). 16. Evolution Ecology Research: 461–473.
- ↑ Musiani, Marco; Leonard, Jennifer A.; Cluff, H. Dean; Gates, C. Cormack; Mariani, Stefano; Paquet, Paul C.; Vilà, Carles; Wayne, Robert K. (2007). "Differentiation of tundra/taiga and boreal coniferous forest wolves: Genetics, coat colour and association with migratory caribou". Molecular Ecology. 16 (19): 4149–70. doi:10.1111/j.1365-294X.2007.03458.x. PMID 17725575.
- ↑ Carmichael, L. E.; Nagy, J. A.; Larter, N. C.; Strobeck, C. (2001). "Prey specialization may influence patterns of gene flow in wolves of the Canadian Northwest". Molecular Ecology. 10 (12): 2787–98. doi:10.1046/j.0962-1083.2001.01408.x. PMID 11903892.
- ↑ Carmichael, L.E., 2006. Ecological Genetics of Northern Wolves and Arctic Foxes. Ph.D. Dissertation. University of Alberta.
- ↑ Geffen, ELI; Anderson, Marti J.; Wayne, Robert K. (2004). "Climate and habitat barriers to dispersal in the highly mobile grey wolf". Molecular Ecology. 13 (8): 2481–90. doi:10.1111/j.1365-294X.2004.02244.x. PMID 15245420.
- ↑ Pilot, Malgorzata; Jedrzejewski, Wlodzimierz; Branicki, Wojciech; Sidorovich, Vadim E.; Jedrzejewska, Bogumila; Stachura, Krystyna; Funk, Stephan M. (2006). "Ecological factors influence population genetic structure of European grey wolves". Molecular Ecology. 15 (14): 4533–53. doi:10.1111/j.1365-294X.2006.03110.x. PMID 17107481.
- 1 2 3 Hofreiter, Michael; Barnes, Ian (2010). "Diversity lost: Are all Holarctic large mammal species just relict populations?". BMC Biology. 8: 46. doi:10.1186/1741-7007-8-46. PMC 2858106
. PMID 20409351.
- ↑ Flower, Lucy O.H.; Schreve, Danielle C. (2014). "An investigation of palaeodietary variability in European Pleistocene canids". Quaternary Science Reviews. 96: 188–203. doi:10.1016/j.quascirev.2014.04.015.
- 1 2 Perri, Angela (2016). "A wolf in dog's clothing: Initial dog domestication and Pleistocene wolf variation". Journal of Archaeological Science. 68: 1–4. doi:10.1016/j.jas.2016.02.003.
- 1 2 Intergovernmental Panel on Climate Change (UN). "IPCC Fourth Assessment Report: Climate Change 2007 – Palaeoclimatic Perspective". The Nobel Foundation.
- 1 2 Clark, P. U.; Dyke, A. S.; Shakun, J. D.; Carlson, A. E.; Clark, J.; Wohlfarth, B.; Mitrovica, J. X.; Hostetler, S. W.; McCabe, A. M. (2009). "The Last Glacial Maximum". Science. 325 (5941): 710–4. doi:10.1126/science.1172873. PMID 19661421.
- ↑ Elias, Scott A.; Short, Susan K.; Nelson, C. Hans; Birks, Hilary H. (1996). "Life and times of the Bering land bridge". Nature. 382 (6586): 60–63. doi:10.1038/382060a0.
- ↑ Germonpré, Mietje; Sablin, Mikhail V.; Stevens, Rhiannon E.; Hedges, Robert E.M.; Hofreiter, Michael; Stiller, Mathias; Despre´s, Viviane R. (2009). "Fossil dogs and wolves from Palaeolithic sites in Belgium, the Ukraine and Russia: osteometry, ancient DNA and stable isotopes". Journal of Archaeological Science. 36 (2): 473–490. doi:10.1016/j.jas.2008.09.033.
- 1 2 Fox-Dobbs, K.; Leonard, J. A.; Koch, P. L. (2008). "Pleistocene megafauna from eastern Beringia: Paleoecological and paleoenvironmental interpretations of stable carbon and nitrogen isotope and radiocarbon records". Palaeogeography, Palaeoclimatology, Palaeoecology. 261 (1–2): 30–46. doi:10.1016/j.palaeo.2007.12.011.
- ↑ Rozell, Ned (October 3, 2012). "Mystery wolf didn't survive in Alaska". Geophysical Institute, University of Alaska – Fairbanks. Retrieved February 19, 2015.
- ↑ DeSantis, L.R.G., Schubert, B.W., Schmitt-Linville, E., Ungar, P.S., Donohue, S.L. and Haupt, R.L. 2015. Dental microwear textures of carnivorans from the La Brea Tar Pits, California and potential extinction implications. Science Series, Natural History Museum of Los Angeles County, 42: 37-52. [www.nhm.org/site/sites/default/files/pdf/contrib_science/lacm-42.pdf]
- 1 2 3 4 5 6 Meachen, Julie A.; Brannick, Alexandria L.; Fry, Trent J. (2016). "Extinct Beringian wolf morphotype found in the continental U.S. Has implications for wolf migration and evolution". Ecology and Evolution. doi:10.1002/ece3.2141.
- 1 2 Baryshnikov, Gennady F.; Mol, Dick; Tikhonov, Alexei N (2009). "Finding of the Late Pleistocene carnivores in Taimyr Peninsula (Russia, Siberia) with paleoecological context" (PDF). Russian Journal of Theriology. Russian Journal of Theriology. 8 (2): 107–113. Retrieved December 23, 2014.
- ↑ Germonpré, Mietje; Sablin, Mikhail V.; Després, Viviane; Hofreiter, Michael; Lázničková-Galetová, Martina; Stevens, Rhiannon E.; Stiller, Mathias (2013). "Palaeolithic dogs and the early domestication of the wolf: A reply to the comments of Crockford and Kuzmin (2012)". Journal of Archaeological Science. 40: 786–792. doi:10.1016/j.jas.2012.06.016.
- ↑ Vila, C; Amorim, I. R.; Leonard, J. A.; Posada, D; Castroviejo, J; Petrucci-Fonseca, F; Crandall, K. A.; Ellegren, H; Wayne, R. K. (1999). "Mitochondrial DNA phylogeography and population history of the grey wolf canis lupus". Molecular Ecology. 8 (12): 2089–103. doi:10.1046/j.1365-294x.1999.00825.x. PMID 10632860. Refer Table 1
- ↑ Lacelle, D., B. Lauriol, G. Zazula, B. Ghaleb, N. Utting, and I. D. Clark. 2013. Timing of advance and basal condition of the Laurentide Ice Sheet during the last glacial maximum in the Richardson Mountains, NWT. Quatern. Res. 80:274–283.
- ↑ Shapiro, B., A. J. Drummond, A. Rambaut, M. C. Wilson, P. E. Matheus, A. V. Sher, et al. 2004. Rise and fall of the Beringian steppe bison. Science 306:1561–1565.
- ↑ Heintzman, Peter D.; Froese, Duane; Ives, John W.; Soares, André E. R.; Zazula, Grant D.; Letts, Brandon; Andrews, Thomas D.; Driver, Jonathan C.; Hall, Elizabeth; Hare, P. Gregory; Jass, Christopher N.; MacKay, Glen; Southon, John R.; Stiller, Mathias; Woywitka, Robin; Suchard, Marc A.; Shapiro, Beth (2016). "Bison phylogeography constrains dispersal and viability of the Ice Free Corridor in western Canada". Proceedings of the National Academy of Sciences: 201601077. doi:10.1073/pnas.1601077113.
- ↑ Meachen, J. A.; Samuels, J. X. (2012). "Evolution in coyotes (Canis latrans) in response to the megafaunal extinctions". Proceedings of the National Academy of Sciences. 109 (11): 4191–6. doi:10.1073/pnas.1113788109. PMID 22371581.
- ↑ "Coyotes "Shrank," Wolves Did Not, After Last Ice Age and Megafaunal Extinctions". National Science Foundation. February 27, 2012. Retrieved December 20, 2014.