Carbon nanotube metal matrix composites
Part of a series of articles on |
Nanotechnology |
---|
Impact and applications |
Nanomaterials |
Molecular self-assembly |
Nanoelectronics |
Nanometrology |
Molecular nanotechnology |
|
Part of a series of articles on |
Nanomaterials |
---|
![]() |
Carbon nanotubes |
Other fullerenes |
Nanoparticles |
|
Carbon nanotube metal matrix composites (CNT-MMC) are an emerging class of new materials that are being developed to take advantage of the high tensile strength and electrical conductivity of carbon nanotube materials. Critical to the realization of CNT-MMC possessing optimal properties in these areas are the development of synthetic techniques that are (a) economically producible, (b) provide for a homogeneous dispersion of nanotubes in the metallic matrix, and (c) lead to strong interfacial adhesion between the metallic matrix and the carbon nanotubes. Since the development of CNT-MMC is still in the research phase, the current focus is primarily on improving these latter two areas.
Carbon nanotubes reinforced metal matrix composites production methods
According to the new production systems, Carbon nanotubes reinforced metal matrix composites (CNT-MMC) may produce several different methods. These production methods are: [1] [2]
Powder metallurgy Route Technics
- Conventional Sintering
- Hot Pressing [3]
- Spark Plasma Sintering
- Deformation Processing
- Hot Extrusion [4]
- Semi-solid Powder Processing [5]
Electrochemical Routes(for non-structural applications)
- Electro-deposition
- Electroless Deposition
- Plasma Spraying
- HVOF Spraying
- Cold Kinetic Spraying
Melt Processing
- Casting
- Melt Infiltration
Novel Techniques
- Molecular Level Mixing
- Sputtering
- Sandwich Processing
- Torsion/Friction Processing
- CVD and PVD (Physical vapor deposition)
- Nanoscale Dispersion
- Pulsed laser deposition
Indigenous techniques
- Such as molecular level mixing (in which CNTs are dispersed into a metal-salt bath, forming a metal-CNT precursor).
- Powder metallurgy techniques
Sintering is one of the oldest method in production technics and used to produce density-controlled materials and components from metal or ceramic powders by applying thermal energy. [6] Synthesis and sintering of nanocrystalline ceramic powders have attracted much attention due to their promising properties.[7] The high active surface area of nanopowders results in lowering sintering temperature relative to coarser powders. Although low temperature sintering suppresses the grain growth, high density of interfaces and grain boundaries in nanocrystalline powders leads to accelerated grain growth during sintering. [8]
- Conventional Sintering is the simplest method for producing CNT metal matrix composite compacts.The CNTs and metal powders are mixed by a process of mechanical alloying/blending and then are compressed to form a green compact, which is then sintered to get the final product. Metallic compacts are subject to oxidation as compared to ceramics and hence the sintering has to be done in an inert atmosphere or under vacuum.[9] One major drawback of this processing route is the inability to tailor the CNT distribution within the metallic matrix.
- Microwave sintering is one of them and fundamentally different from conventional sintering.In microwave sintering process,the material is heated internally and volumetrically unlike in a conventional process where heat originates from an external heating source.Sintering cycle time for microwave sintering is much shorter as compared with the conventional sintering cycle.[10]
- Spark plasma sintering is a quite new technique which takes only a few minutes to complete a sintering process compared to conventional sintering which may take hours or even days for the same.High sintering rate is possible in SPS since high heating rates can be easily attained due to internal heating of the sample as oppsed to external heating seen in case of conventional sintering.For conventional sintering usually a green compact needs to be prepared externally using a suitable die and hydraulic machine for applying the necessary pressure.In SPS the powder is directly fed into the graphite dies and the die is enclosed with suitable punches.All types of materials, even those difficult to densify can be easily sintered in SPS.Due to advantage of high heating rate and less holding time, SPS can restrict the unwanted sintering reactions in highly reactive systems as opposed to conventional sintering and hence formation of undesirable product phases can be avoided.[11]
- Semi-solid Powder Processing (SPP) is a unique method that fabricates composites materials with powder mixtures in the semi-solid states. Starting with metal-CNT powder mixture, the metal powder is heated to the semi-solid state, and pressure is applied to form the metal matrix composites. This method features many advantages such as simple and fast process and flexible property tailoring.[12]
- Carbon nanotube dispersing and CNT breakage during mixing
One common method to disperse the CNT into the metal matrix is mechanical alloying. However, many researchers reported the length reduction and damage of CNTs during mechanical alloying process.[13]
Mechanical Properties
Carbon nanotubes are the strongest and stiffest materials yet discovered in terms of tensile strength and elastic modulus respectively. This strength results from the covalent sp2 bonds formed between the individual carbon atoms. Multi-walled carbon nanotube was tested to have a tensile strength of 63 gigapascals (GPa).[14] Further studies, conducted in 2008, revealed that individual CNT shells have strengths of up to ~100 GPa, which is in good agreement with quantum/atomistic models.[15] Since carbon nanotubes have a low density for a solid of 1.3 to 1.4 g/cm3,[16] its specific strength of up to 48,000 kN·m·kg−1 is the best of known materials, compared to high-carbon steel's 154 kN·m·kg−1. CNTs are not nearly as strong under compression. Because of their hollow structure and high aspect ratio, they tend to undergo buckling when placed under compressive, torsional, or bending stress.[17]
Material | Young's modulus (TPa) | Tensile strength (GPa) | Elongation at break (%) |
---|---|---|---|
SWNTE | ~1 (from 1 to 5) | 13–53 | 16 |
Armchair SWNTT | 0.94 | 126.2 | 23.1 |
Zigzag SWNTT | 0.94 | 94.5 | 15.6–17.5 |
Chiral SWNT | 0.92 | ||
MWNTE | 0.2[14]–0.8[22]–0.95[14] | 11[14]–63[14]–150[22] | |
Stainless steelE | 0.186[23]–0.214[24] | 0.38[23]–1.55[24] | 15–50 |
Kevlar–29&149E | 0.06–0.18[25] | 3.6–3.8[25] | ~2 |
EExperimental observation; TTheoretical prediction
Potential applications
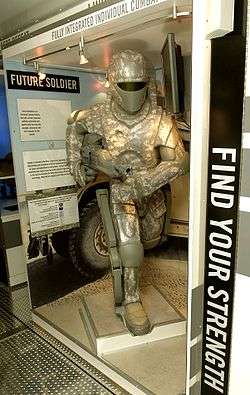

Nanonetwork
Nanonetworks are expected to expand the capabilities of single nanomachines both in terms of complexity and range of operation by allowing them to coordinate, share and fuse information. CNT metal matrix composites enable new applications of nanotechnology in the military technology and industrial and consumer goods applications.
Nanorobotics
Nanomachines are largely in the research-and-development phase,[26] but some primitive molecular machines have been tested. An example is a sensor having a switch approximately 1.5 nanometers across, capable of counting specific molecules in a chemical sample. The first useful applications of nanomachines might be in medical technology,[27] which could be used to identify and destroy cancer cells.[28][29] Another potential application is the detection of toxic chemicals, and the measurement of their concentrations, in the environment.In addition CNT-MM composite will be the main material for the military robots, especially to strength the robot soldier's armours.
Future Soldier
Today's militaries often use high-quality helmets made of ballistic materials such as Kevlar and Aramid, which offer improved protection. Some helmets also have good non-ballistic protective qualities, though many do not.[30] Non-ballistic injuries may be caused by many things, such as concussive shock waves from explosions, physical attacks, motor vehicle accidents, or falls.[31] Another application for the future soldier is powered exoskeleton system. Powered exoskeleton, also known as powered armor, or exoframe, is a powered mobile machine consisting primarily of an exoskeleton-like framework worn by a person and a power supply that supplies at least part of the activation-energy for limb movement.Powered exoskeletons are designed to assist and protect the soldiers and officers.Currently MIT is working on combat jackets that use CNT fibers to stop bullets and to monitor the condition of the wearer.[32]
Advanced Modular Armor Protection
Advanced Modular Armor Protection (AMAP) is modular composite armour concept, developed by the German company IBD Deisenroth Engineering. According to IBD AMAP is a 4th generation composite armour, making use of nano-ceramics and modern steel alloy technologies.[33] AMAP is making use of new advanced steel alloys, Aluminium-Titanium alloys, nanometric steels, ceramics and nano-ceramics. The new high-hardened steel needs 30% less thickness to offer the same protection level as ARMOX500Z High Hard Armour steel.[33] While Titanium requires only 58% as much weight as rolled homogeneous armour (RHA) for reaching the same level of protection, Mat 7720 new, a newly developed Aluminium-Titanium alloy, needs only 38% of the weight.[33] That means that this alloy is more than twice as protective as RHA of the same weight.
AMAP is also making use of new nano-ceramics, which are harder and lighter than current ceramics, while having multi-hit capability. Normal ceramic tiles and a liner backing have a mass-efficiency (EM) value of 3 compared to normal steel armour, while it fulfills STANAG 4569. The new nano-crystalline ceramic materials should increase the hardness compared to current ceramics by 70% and the weight reduction is 30%, therefore the EM value is larger than 4.[33] Furthermore, the higher fracture toughness increases the general multi-hit capability. Some AMAP-modules might consist of this new ceramic tiles glued on a backing liner and overlaid by a cover, a concept which is also used by MEXAS.[34] Lightweight SLAT armour is also part of the AMAP family.
Nano Armour
The TK-X (MBT-X) project, the new Type 10 main battle tank design use composition of modular components of nano-crystal steel (nor Triple Hardness Steel), Modular ceramic composite armor, partly reinforced MMC and Light weight upper armor.
Materiomics
Materiomics is defined as the study of the material properties of natural and synthetic materials by examining fundamental links between processes, structures and properties at multiple scales, from nano to macro, by using systematic experimental, theoretical or computational methods and refers to the study of the processes, structures and properties of materials from a fundamental, systematic perspective by incorporating all relevant scales, from nano to macro, in the synthesis and function of materials and structures. The integrated view of these interactions at all scales is referred to as a material's materiome.
Materiomics includes the study of a broad range of materials, which includes metals, ceramics and polymers as well as biological materials and tissues and their interaction with synthetic materials. Materiomics finds applications in elucidating the biological role of materials in biology, for instance in the progression and diagnosis or the treatment of diseases. Others have proposed to apply materiomics concepts to help identify new material platforms for tissue engineering applications, for instance for the de novo development of biomaterials. Materiomics might also hold promises for nanoscience and nanotechnology, where the understanding of material concepts at multiple scales could enable the bottom-up development of new structures and materials or devices, including biomimetic and bioinspired structures.
Nanotough
Nanotough is to obtain a deeper understanding of the interfacial structure of nanocomposites within a polyolefin matrix and thus use nanoparticles like nanoclay to turn upside down the construction of a number of well-known products, where today metals or plastics are used in for example cars or aircraft. The project will enable realization of the great performance potential of these materials through development of novel multiphase and hybrid nanocomposites.
Nanotough project aims to improve the stiffness of polyolefin nanocomposites while not only maintaining but also improving the toughness of the matrix considerably. The technical objective is to optimize and, through novel interface design, to develop new cost effective hybrid (nanofiller-fibre) nanocomposites as an alternative to heavily filled polymers and expensive engineering polymers and fulfill industry requirements for high performance materials in high tech applications. [35]
References
- ↑ S. R. Bakshi, D. Lahiri, and A. Argawal, Carbon nanotube reinforced metal matrix composites - A Review, International Materials Reviews, vol. 55, p.41 (2010), http://web.eng.fiu.edu/agarwala/PDF/2010/12.pdf
- ↑ Arvind Agarwal, Srinivasa Rao Bakshi,Debrupa Lahiri, Carbon nanotubes reinforced metal matrix composites, CRC Press, p. (4-5-6-7-8), http://www.crcpress.com
- ↑ Suarez, S.; Lasserre, F.; Prat, O.; Mücklich, F. (2014). "Processing and interfacial reaction evaluation in MWNT/Ni bulk composites". Physica Status Solidi A. 211: 1555–1561. doi:10.1002/pssa.201431018.
- ↑ Mortazavi Majid, G. H. Majzoobi, Golikand A. Noozad, A. Reihani, S. Z. Mortazavi, M. S. Gorji, 2012 Fabrication and mechanical properties of MWCNTs-reinforced aluminum composites by hot extrusion ,Rare Metals, Volume 31, Issue 4, pp 372-378, doi 10.1007%2Fs12598-012-0523-6
- ↑ Wu, Yufeng; Gap; Kim, Yong (2011). "Carbon nanotube reinforced aluminum composite fabricated by semi-solid powder processing". Journal of Materials Processing Technology. 211 (8): 1341–1347. doi:10.1016/j.jmatprotec.2011.03.007.
- ↑ Suk-Joong, L. Kang Sintering -Densification, Grain Growth and Microstructure, Elsevier Butterworth-Heinemann, 2005, ISBN 0-7506-6385-5, p.3
- ↑ V.V. Srdic, M. Winterer, and H. Hahn, Sintering Behavior of Nanocrystalline Zirconia Doped with Alumina Prepared by Chemical Vapor Synthesis, J. Am. Ceram. Soc., 83, 1853-60 (2000).
- ↑ Driver, J. H. (2004). "Stability of Nanostructured Metals and Alloys". Scripta Materialia. 51: 819–823. doi:10.1016/j.scriptamat.2004.05.014.
- ↑ Arvind Agarwal, Srinivasa Rao Bakshi, Debrupa Lahiri, Carbon nanotubes reinforced metal matrix composites , CRC Press, p.20, http://www.crcpress.com,
- ↑ A. Mondal, A. Upadhaya, D. Agrawal Microwave and conventional sintering of premixed and prealloyed tungsten heavy alloys, 2008 MS&T08, p.2502 (2008)
- ↑ Materials and Metallurgical Engineering Indian Institute of Technology Kanpur, Spark Plasma Sintering,http://www.iitk.ac.in/biomaterialslab/Spark%20Plasma%20Sintering.pdf, p.2
- ↑ Wu, Yufeng; Yong Kim, Gap; Russell, Alan (2012). "Effects of mechanical alloying on an Al6061–CNT composite fabricated by semi-solid powder processing". Materials Science and Engineering: A. 538: 164–172. doi:10.1016/j.msea.2012.01.025.
- ↑ Wu, Yufeng; Yong Kim, Gap; Russell, Alan (2012). "Mechanical alloying of carbon nanotube and Al6061 powder for metal matrix composites". Materials Science and Engineering: A. 532: 558–566. doi:10.1016/j.msea.2011.10.121.
- 1 2 3 4 5 Yu, Min-Feng; Lourie, Oleg; Dyer, Mark J.; Moloni, Katerina; Kelly, Thomas F.; Ruoff, Rodney S. (28 January 2000). "Strength and Breaking Mechanism of Multiwalled Carbon Nanotubes Under Tensile Load". Science. 287 (5453): 637–640. Bibcode:2000Sci...287..637Y. doi:10.1126/science.287.5453.637. PMID 10649994.
- ↑ Peng, B.; Locascio, M.; Zapol, P.; Li, S.; Mielke, S. L.; Schatz, G. C.; Espinosa, H. D. (2008). "Measurements of near-ultimate strength for multiwalled carbon nanotubes and irradiation-induced crosslinking improvements". Nature Nanotechnology. 3: 626–631. doi:10.1038/nnano.2008.211. PMID 18839003.
- ↑ Collins, Philip G. (2000). "Nanotubes for Electronics" (PDF). Scientific American: 67–69. Archived from the original (PDF) on 2008-06-27.
- ↑ Jensen, K.; Mickelson, W.; Kis, A.; Zettl, A. (2007). "Buckling and kinking force measurements on individual multiwalled carbon nanotubes". Phys. Rev. B. 76: 195436. doi:10.1103/physrevb.76.195436.
- ↑ Belluci, S. (19 January 2005). "Carbon nanotubes: physics and applications". Physica Status Solidi (c). 2 (1): 34–47. Bibcode:2005PSSCR...2...34B. doi:10.1002/pssc.200460105.
- ↑ Chae, Han Gi; Kumar, Satish (26 January 2006). "Rigid Rod Polymeric Fibers". Journal of Applied Polymer Science. 100 (1): 791–802. doi:10.1002/app.22680.
- ↑ Meo, Michele; Rossi, Marco (3 February 2006). "Prediction of Young's modulus of single wall carbon nanotubes by molecular-mechanics-based finite element modelling". Composites Science and Technology. 66 (11–12): 1597–1605. doi:10.1016/j.compscitech.2005.11.015.
- ↑ Sinnott, Susan B.; Andrews, Rodney (July 2001). "Carbon Nanotubes: Synthesis, Properties, and Applications". Critical Reviews in Solid State and Materials Sciences. 26 (3): 145–249. Bibcode:2001CRSSM..26..145S. doi:10.1080/20014091104189.
- 1 2 Demczyk, B.G.; Wang, Y; Cumings, J; Hetman, M; Han, W; Zettl, A; Ritchie, R (2002). "Direct mechanical measurement of the tensile strength and elastic modulus of multiwalled carbon nanotubes". Materials Science and Engineering A. 334 (1–2): 173–178. doi:10.1016/S0921-5093(01)01807-X.
- 1 2 Australian Stainless Steel Development Association (ASSDA) – Properties of Stainless Steel
- 1 2 Stainless Steel – 17-7PH (Fe/Cr17/Ni 7) Material Information Archived July 19, 2011, at the Wayback Machine.
- 1 2 H. D. Wagner (2002). "Reinforcement". Encyclopedia of Polymer Science and Technology (PDF). John Wiley & Sons. doi:10.1002/0471440264.pst317.
- ↑ Wang, J. (2009). "Can Man-Made Nanomachines Compete with Nature Biomotors?". ACS Nano. 3 (1): 4–9. doi:10.1021/nn800829k. PMID 19206241.
- ↑ Amrute-Nayak, M.; Diensthuber, R. P.; Steffen, W.; Kathmann, D.; Hartmann, F. K.; Fedorov, R.; Urbanke, C.; Manstein, D. J.; Brenner, B.; Tsiavaliaris, G. (2010). "Targeted Optimization of a Protein Nanomachine for Operation in Biohybrid Devices". Angewandte Chemie. 122 (2): 322–326. doi:10.1002/ange.200905200.
- ↑ Patel, G. M.; Patel, G. C.; Patel, R. B.; Patel, J. K.; Patel, M. (2010). "Nanorobot: A versatile tool in nanomedicine". Journal of Drug Targeting. 14 (2): 63–67. doi:10.1080/10611860600612862. PMID 16608733.
- ↑ Wang, J. et al . (2011). "Micromachine Enables Capture and Isolation of Cancer Cells in Complex Media". Angew. Chem. Int. Ed. 50: 4161–4165. doi:10.1002/anie.201100115.
- ↑ Kevlar Pasgt Helmet
- ↑ http://www.operation-helmet.org
- ↑ "MIT Institute For Soldier Nanotechnologies". Web.mit.edu. Retrieved 2010-02-26.
- 1 2 3 4 Michael Rust. "Passive Protection Concepts" (PDF). Archived from the original (PDF) on 8 October 2011. Retrieved 15 December 2010.
- ↑ A graphical rendering from IBD-Deisenroth-Engineering.de called stream_sandwich.wmv
- ↑ About Nanotough at Nanotough.aau.dk