Siletzia
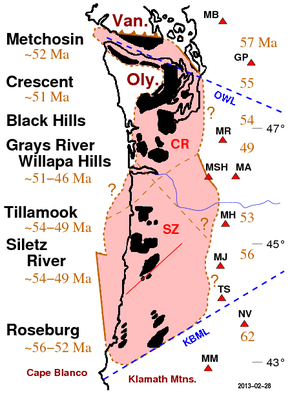
Siletzia is the massive formation of early to middle Eocene epoch marine basalts and interbedded sediments in the forearc of the Cascadia subduction zone; this forms the basement rock under western Oregon and Washington and the southern tip of Vancouver Island.[1] It is now fragmented into the Siletz and Crescent terranes.[2]
Siletzia corresponds geographically to the Coast Range Volcanic Province (or Coast Range basalts),[3] but is distinguished from slightly younger basalts that erupted after Siletzia accreted to the continent and differ in chemical composition.[4] The Siletzia basalts are tholeiitic, a characteristic of mantle-derived magma erupted from a spreading ridge between plates of oceanic crust. The younger basalts are alkalic or calc-alkaline, characteristic of magmas derived from a subduction zone.[5] This change of composition reflects a change from marine to continental volcanism that becomes evident around 48 to 42 Ma (millions of years ago), and is attributed to the accretion of Siletzia against the North American continent.[6]
Various theories have been proposed to account for the volume and diversity of Siletzian magmatism, as well as the approximately 75° of rotation, but the evidence is insufficient to determine Siletzia's origin; the question remains open.[7]
The accretion of Siletzia against the North American continent approximately 50 million years ago (contemporaneous with the initiation of the bend in the Hawaiian-Emperor seamount chain) was a major tectonic event associated with a reorganization of the earth's tectonic plates.[8] This is believed to have a caused a shift in the subduction zone, termination of the Laramide orogeny that was uplifting the Rocky Mountains, and major changes in tectonic and volcanic activity across much of western North America.[9]
Exposures and discovery
The rock of Siletzia has been exposed in various places by tectonic uplift (as around the periphery of the Olympic Mountains), anticlinal folding (such as the Black Hills and Willapa Hills in southwestern Washington), and overthrusting onto other formations (along various faults in central and southern Oregon). These exposures have been variously named the Metchosin Formation of Vancouver Island, the Crescent Formation, Black Hills, and Willapa Hills volcanics of Washington, and the Siletz River Volcanics and Roseburg Formation of Oregon.[10] (See map. The Grays River Volcanics of Washington and Tillamook Volcanics of Oregon are now considered post-Siletz.[11]) Elsewhere Siletzia is covered by younger volcanic and sedimentary deposits.
The discovery of Siletzia began in 1906 with Arnold's description and naming of a small exposure on the north side of the Olympic Peninsula near Port Crescent.[12] Though this exposure is small, he recognized as very likely that much more of it was buried under younger deposits. With recognition that similar rock exposed at other outcrops is part of the same formation, the name is now generally applied to all early and middle Eocene basalts on the Olympic Peninsula and Puget Lowland.[13]
The Metchosin formation at the southern tip of Vancouver Island was described in a series of reports (1910, 1912, 1913, 1917) by Clapp, who recognized it as correlative with the Crescent formation on the other side of the Strait of Juan de Fuca.[14] Weaver recognized that these "Metchosin volcanics" included various Eocene basalts in western Washington and the Oregon Coast Range as far south as the Klamath Mountains.[15] The Siletz River Volcanics was described in 1948 by Snavely and Baldwin after exposures near the Siletz River, Oregon,[16] and the Roseburg and related formations in southern Oregon described in various reports from the 1960s on.[17]
"Siletzia" was coined in 1979 by Irving to describe the full extent of these Eocene basalts and interbedded sedimentary formations.[18]
Extent
The map shows the exposures (black) and inferred near-surface extent (pink) of Siletzia, the latter being what can be detected in the upper crust by aeromagnetic, gravitational, or seismological studies.[19]
There are only two exposed contacts of Siletzia with the older (pre-Cenozoic) North American basement.[20] One is near Roseburg, Oregon, where it is thrust against formations of the Klamath Mountains (discussed below), the other is along the Leech River Fault on the southern end of Vancouver Island, where it has pushed the pre-Cenozoic Pacific Rim formation beneath the Wrangellia Terrane).[21] Everywhere else the contact between Siletzia and the rest of the continent is concealed under younger deposits, especially the Cascade Range volcanics. The contact around the Olympic Mountains is actually the bottom contact with the underlying oceanic sediments, tilted up by the uplift of the Olympics and exposed by erosion of about 10 to 12 km of overlying deposits.[22]
The location of the near-surface contact between the Crescent Formation and the pre-Cenozoic metamorphic basement of the continent — what has been the termed the Coast Range Boundary Fault (CRBF) — is largely uncertain. The Leech River Fault extends southeast past Victoria, B.C. to cross the Strait of Juan de Fuca, possibly connecting with the southeast striking Southern Whidbey Island Fault (SWIF).[23] This extends to the Rattlesnake Mountain Fault Zone (RMFZ), some 25 kilometers east of Seattle, which is believed to be the western edge of the pre-Cenozoic basement. However, gravity data indicates that at this latitude the Crescent Formation (at least near the surface) extends no further east than Seattle.[24]
Further south, near Mount St. Helens, is a similar situation, where the St. Helens Fault Zone (SHZ) is believed to be the eastern edge of the Crescent Formation,[25] but the pre-Cenozoic continental basement is near Mount Rainier. Separating these is the marine sedimentary formation known as the Southern Washington Cascades Conductor (SWCC); it is possible that it was deposited over a fragment of Siletzia.[26] Or not: the oldest parts of the SWCC likely predate Siletzia,[27] and the nature and location of the contact between these two formations is unknown.
In central Oregon, Siletzia forms a platform on which the older, now defunct volcanoes of the Western Cascades rest. The younger High Cascades to the east are believed to rest on sediments that accumulated in the basin between Siletzia and the continent.[28]
In southern Oregon, Siletzia has been thrust against the Mesozoic Klamath Mountains of southern Oregon along the Klamath—Blue Mountain Lineament (KBML).[29] Near Roseburg this contact is exposed at the Wild Safari Fault where the Late Jurassic Dothan Formation has been thrust over the Roseburg Formation.[30]
Off the coast of southern Oregon, the western edge of Siletzia is the Eocene Fulmar fault.[31] This is a strike-slip fault, where part of Siletzia has been split off; the missing piece may be the Yakutat terrane now at the head of the Gulf of Alaska.[32] Further north, the terrane boundary is believed to come ashore near the Columbia River.[33]
The way the Crescent Formation wraps around the Olympic Mountains ("Oly" on the map) may reflect oroclinal bending as a result of being crushed against Vancouver Island.[34] It has also been attributed to loss of the deposits originally overlying the Olympics prior to their uplift,[35] resembling a dome where top and western end has been removed.
Siletzia's actual thickness, and the estimates of that thickness, vary. Under Oregon, the Siletz terrane appears to extend 25 to possibly 35 km into the trough between the subducting Juan de Fuca plate and the edge of the continent, where it is slipping over sediments accumulated in the bottom of the trough.[36] The Crescent terrane (under Washington) is believed to be thinner, from as little as 12 and 22 km under the western and eastern ends of the Strait of Juan de Fuca, but possibly as much as 20 and 35 km thick.[37]
Composition
The various formations of Siletzia are characterized as marine tholeiitic pillow basalts and volcanic breccia, often interbedded with sedimentary layers of continental origin, lying on oceanic crust. These are usually capped by a layer of alkalic volcanics deposited subaerially.[38] All this suggests these formations were initially deposited in an oceanic environment, possibly as seamounts or an island arc.[39]
On the Olympic Peninsula the Blue Mountain unit at the base of the Crescent Formation includes sediments (including large boulders of quartz diorite) of continental origin, showing that the continent was close by;[40] other sediments were eroded from the pre-Cenozoic rock of Vancouver Island and the northern Cascade Range.[41] At the southern end are sediments derived from the Klamath Mountains,[42] while sand of the overlying Tyee Formation has an isotopic composition corresponding to rock of the Idaho Batholith.[43]
Age
Eruption of the Siletzia basalts has been placed roughly in the late Paleocene through the mid Eocene; more specific dates have been difficult to obtain and somewhat variable. Early K-Ar (potassium-argon) and 40Ar-39Ar (argon-argon) radiometric dating by Duncan gave dates of 57 and 62 Ma (million years ago) to the northern and southern ends, and a date of 49 Ma for the Grays River volcanics near the center of Siletzia.[44] This is suggestive of a spreading ridge (as previously noted by McWilliams 1980), and has been a strong constraint on models of how Siletzia formed. Other researchers have since found younger dates (50-48 Ma) for the Crescent basalts, removing much of the age symmetry.[45]
More recent dating based on 40Ar-39Ar, U-Pb (uranium-lead), and coccoliths shows a narrower range of ages from 56 Ma in the south to 50 or 49 Ma in the north.[46]
Size
Siletzia is massive: over 400 miles (600 kilometers) long, almost half that much across (and likely further at depth). The original deposits were from 16 to 35 kilometers thick.[47] Weaver, reckoning a minimal thickness of only 3,000 feet, still estimated "nearly 10,000 cubic miles of rock";[48] he put the total volume to be as great, if not greater, than the better known Columbia River Basalts.[49] Snavely et al., recognizing at least 10,000 feet of thickness, and as much as 20,000 feet under eruptive centers, estimated the volume to be in excess of 50,000 cubic miles (over 200,000 km3).[50] Duncan (1982) estimated around 250,000 km3 (about 60,000 cubic miles), which exceeds the volume of most continental rift zones, and some flood basalt provinces.[51] A recent estimate put the volume at 2 million cubic km.[52]
Paleorotation

When lava solidifies it retains an imprint of the earth's magnetic field, thus recording how it was oriented. Measurements of such paleomagnetic fields in the Oregon Coast Range show rotations of 46 to 75°, all of it following the presumed accretion to the continent (alternately, formation) of the Siletz terrane at about 50 Ma. These rotations are all clockwise, and show a strong correlation with the age of the rock: about one and a half degrees of rotation per million years.[53] These paleomagnetic rotations and other evidence show that Siletzia — or the part of it constituting the Siletz terrane ("SZ" on map, above), from the Klamath Mountains to the Columbia River — has rotated clockwise as a single, coherent block.[54]
Did Siletzia pivot about its northern end or southern end? This question has attracted considerable attention, with the evidence now favoring a northern pivot.[55] A key piece of evidence is that the Crescent Formation is laid over sediments (the Blue Mountain unit) derived from the continent, including boulders of quartz diorite some 65 million years old; this requires the Crescent Formation to have formed close to the continent.[56]
This model has Siletzia forming on the continental margin along what is now the Olympic-Wallowa Lineament (OWL; a zone of topographical features of unknown age and tectonic significance), with the southern end of Siletzia and the Klamath Mountains (joined to Siletzia) near the Idaho Batholith in central Idaho. Further evidence for this comes from the sand of the Tyee Formation that overlie the Roseburg Formation. Not only does this sand have the same isotopic composition of rock in the Idaho Batholith (and of sand now coursing down the Snake and Columbia Rivers), but it appears to have not been transported very far from its source. This implies that the Tyee Formation was much closer to the Idaho Batholith when it was deposited, and subsequently rotated away.[57] Geodetic surveys show that the region continues to rotate, likely as a result of extension of the Basin and Range Province[58] and asthenospheric flow around the southern edge of the subducting Juan de Fuca plate.[59]
North of the Columbia River, matters are more complicated. First, in southwestern Washington there is only half as much rotation as seen in rocks of similar age in Oregon. This is the basis for believing the Crescent terrane has broken from the Siletz terrane (perhaps because they formed on different oceanic plates[60]), and undergone a different rotational history.[61] Second, in Washington there is more variation in the amount of rotation and more faulting, which has led to a speculation that the Crescent terrane has broken up into eight or nine crustal blocks.[62]
At Bremerton, on the east side of the Olympics, the measured rotations are less, and within the statistical error bounds of being zero; while further north, near Port Townsend, the rotation is slightly counter-clockwise.[63] On Vancouver Island the paleorotations are counter-clockwise, and other evidence shows that the tip of the island has been bent, presumably as a result of the collision of Siletzia.[64] The northwestern tip of the Olympic Peninsula also shows counter-clockwise rotation, of around 45 degrees. This raises a question of how much of the arcuate shape of the Crescent Formation is due to loss of material from the center after uplift by the Olympic Mountains, and how much reflects oroclinal bending.[65]
Origin
Siletzia's origin is not yet determined, and (as of 2011) lacks a definite answer.[66] Theories are still being developed, and even the details the theories depend on "have remained enigmatic".[67] Following are several of the most notable models.
Models of how Siletzia formed are of two general types:[68] (1) Formation well offshore (possibly as seamounts, like the Hawaiian-Emperor seamount chain, or a hotspot at a spreading ridge, like Iceland) and then accretion to the continent; (2) formation inshore, on or near the continental margin (perhaps as a result of transcurrent extension, or of a slab window). All current models then have Siletzia rifting away from the continental edge about a northern pivot.[69] Studies of Siletzia's origins have generally focused on accounting for two principal observations: the large paleorotation (described above), and the voluminous output (over 50,000 cubic miles, exceeding the volume of most continental rift zones, and some flood basalt provinces[70]). Accounting for the observed volumes of basalt requires an enhanced magmatic source, for which most models invoke either the presence of the Yellowstone hotspot, or slab windows.[71] The latter would have resulted from the subduction of the Farallon—Kula (or possibly Farallon—Resurrection) spreading ridge. The relation with the Kula-Farallon spreading ridge is an important element in all models, though its location through that epoch is not well determined.[72]
Simpson & Cox 1977: Two models
Seeking to explain the observed clockwise paleorotation, and noting that Siletzia appeared to have rotated as a rigid block, Simpson & Cox (1977) proposed two models. First was rotation about a southern pivot in contact with the Klamath Mountains. This has various problems, especially because at the northern end sediments and even boulders from the continent are found at the base of the Crescent Formation, showing that it was near the continent from the beginning.[73] In the second model (subsequently refined by Hammond 1979), Siletzia was originally adjacent to the Olympic-Wallowa Lineament, then rifted from the continent and rotated about a northern pivot near the Olympic Peninsula. Because sediments also show the Klamaths in close contact from the start, this requires the Klamaths to have moved with Siletzia. Originally there were conflicts in the understanding of when the Klamaths moved, and with the age and amount of rotation of the Clarno Formation in central Oregon. These were largely cleared up in a study of the Clarno Formation by Grommé et al. (1986) and illustrated with a palinspastic reconstruction as of 38 Ma.
Offshore model: A captured island chain?
An early and widely cited paper by Duncan (1982) (drawing on features of the fairly new theory of plate tectonics) exemplifies the off-shore or "seamount" type of models. It featured a set of radiometrically determined (K-Ar and 40Ar-39Ar) ages that were younger in the center (for the Grays River volcanics) and older at the ends. This dihedrally symmetric age progression strongly suggested the pattern seen at spreading ridges, where the older rock is carried away on both sides from where the new rock erupts. Duncan considered five models (but none involving rifting or ridge subduction),[74] favoring one where a hotspot — presumably the Yellowstone hotspot — intersected the Farallon-Kula spreading ridge (such as at Iceland) to generate a chain of islands. These islands were then accreted to the continent as the underlying oceanic crust was subducted.
This study has been criticized on multiple grounds, particularly regarding the ages. Duncan himself noted that measurement of the northern ages may have been affected by loss of argon due to low-grade metamorphism, and that there might be bias in respect of stratigraphic position.[75] The latter was demonstrated by a recent study that showed, on the basis of geochemistry, that the Grays River volcanics followed the Siletzia eruptions,[76] and thus are not representative of the initial phase of Siletz magmatism. Recent dating also shows a more monotonic trend of south to north age progression ("younging").[77]
The range of the original ages was also a problem, as the rate of Kula-Farallon spreading over that time would produce a chain of seamounts much longer than observed, and too far away from the continent to explain the continentally derived sediments.[78] This objection is attenuated somewhat in that the newer ages show a smaller range of ages.[79]
Inshore models
Various models have Siletzia forming inshore, on or near the continental margin. While all current models have Siletzia rifting away from the continent after accretion or formation, a subclass of "rifted" models consider the rifting to have caused the Siletzia eruptions.
Wells et al. 1984 proposed that the Siletzia basalts might have "leaked" through transform faults (perpendicular to a spreading ridge) during changes in direction of the tectonic plates. The size of these eruptions and their location in this region is attributed to proximity to the Yellowstone hotspot.[80] This "leaky transform" theory seems to be largely rejected, likely because the plate motion model it was based on was shown to be faulty.[81]
Wells, et al., alternately suggested that as a terrane at the margin of the continent was pushed over the Yellowstone hotspot, it was rifted away from the continent by the upwelling magma, which then formed the Siletzia basalts.[82] This idea was further developed by Babcock et al. (1992), who suggested rifting might have been initiated by a change in plate direction, or by kinematic effects as the Kula-Farallon ridge migrated along the continental margin. One such effect is the formation of a slab window (or slab gap) which would allow increased upwelling of magma.[83]
Slab windows
That spreading ridges could be subducted was recognized early in the development of plate tectonic theory, but there was little consideration of the ensuing effects. In the 1980s came realization that the magma welling up from the asthenosphere through the subducted ridge would not reach seawater, and thus not be quenched to form rock and close the gap. Continued spreading would lead to a widening gap or "window" in the subducting plate through which there could be increased flow of magma[84] The implications of this for Siletzia were first shown by Thorkelson & Taylor (1989) and Babcock et al. (1992) (following the pioneering work by Dickinson & Snyder 1979[85]). Breitsprecher et al. (2003) subsequently identified the fan-shaped wake of volcanics of distinctive geochemistry left by the widening Kula-Farallon slab window across northeastern Washington and into Idaho.
Madsen et al. (2006) showed that most of the Eocene and subsequent magmatism from Alaska to Oregon "is explainable in terms of ridge subduction and slab window tectonics."[86] That is, a slab window — and a single subducted ridge can give rise to multiple slab windows — can provide adequate magmatism without having to invoke a hotspot (mantle plume). (So much so that it has been suggested that the Yellowstone hotspot may have been initiated by a slab window.[87]) Mantle plumes and slab windows both feature voluminous magmatism; the main difference is that slab windows would form only where the spreading ridge is subducted. This implies formation at the continental margin, and then rifting, in the manner of the second class of models.
Gulf of Alaska
Any model of the origin of Siletzia must account for interactions with plate boundaries that were being subducted under North America through the Eocene. Early studies were plagued by indeterminate locations for these boundaries, particularly of the Kula-Farallon (K-F) spreading ridge: basalts at the head of the Gulf of Alaska (along the Alaska panhandle) have ages and compositions corresponding to the Siletz volcanics, suggesting that the K-F ridge was offshore of the Yukon at the same time it was offshore of Washington. This can be resolved by assuming that by about 56 Ma the eastern part of the Kula plate had broken away to form the Resurrection plate, with the new Kula-Resurrection (K-R) spreading ridge running up the Gulf of Alaska towards Kodiak Island, and the former K-F (now R-F) ridge reaching Washington.[88] Subduction of this plate under western Canada was rapid, and it disappeared entirely with subduction of the K-R ridge about 50 Ma.[89]
This scenario then permits rapid transport north of crustal blocks such as the Yakutat terrane. Now lying southeast of Cordova at the head of the Gulf of Alaska, paleomagnetic evidence indicates it was formed at a latitude corresponding to Oregon or northern California.[90] Similarly, certain schists on Baranof Island are believed to have been contiguous with the Leech River Schists (Leech River Complex) on Vancouver Island around 50 Ma, and subsequently transported northward with other elements of the Chugach-Prince William terrane.[91]
After accretion: 50-42 Ma
Whether formed far offshore as seamounts, or close inshore by a slab window, the Siletzian basalts were laid down on a subducting oceanic plate: the Siletz terrane on the Farallon plate, and the Crescent terrane most likely on the adjoining Resurrection plate (previously broken away from the Kula plate, which had previously broken away from the Farallon plate). In both cases the Siletzia mass was drawn toward the subduction zone, which possibly ran diagonally across what is now Washington, approximately at the position of the Olympic-Wallowa Lineament.[92] However, Siletiza was too big to be subducted, and it accreted to the continent. Accretion is sometimes called "docking," but is more akin to a collision: various peripheral structures are first folded or crushed, then the main structures are deformed when they come into contact, and various parts get pushed over other parts, all this playing out over several million years. To the extent that the accretion of Siletzia to North America can be given a definite date most studies give it as about 50 Ma.[93] This date has added significance as it is also the start of a change in direction of the Pacific plate, as seen in the bend in the Hawaiian-Emperor seamount chain, and also a change in the Pacific Northwest from compressional to extensional tectonics.[94] This may also be when the last of the Resurrection plate was subducted under British Columbia.[95] Initiation of the north-striking right-lateral Straight Creek Fault at ~48 Ma[96] likely resulted from strain accumulated during the accretion of Siletzia.
As Siletzia accreted it also jammed the existing subduction zone, halting subduction of the Farallon plate. This terminated the Laramide orogeny that had been uplifting the Rocky Mountains, and triggered the ignimbrite sweep, a wave of large-volume silicic magmatism that swept over much of western North America between 50 and 20 Ma.[97] This undoubtedly affected the enigmatic and controversial Challis Arc (stretching from southeastern British Columbia to the Idaho Batholith, roughly parallel with the Olympic-Wallowa Lineament), but the details of this are unknown.[98]
Subduction, having ceased at the existing zone, eventually reinitiated further to the west as the current Cascadia subduction zone.[99] Volcanism from the new subduction zone (such as the Grays River Volcanics[100] and Northcraft Volcanics[101]) reached the surface about 42 Ma, thereby initiating the rise of the ancestral Cascade Range.[102]
Several other significant events occurred around 42 Ma, including cessation of metamorphism of the Leech River Schists[103] (resulting from the Metchosin/Crescent Formation being thrust under Vancouver Island) and the end of strike-slip motion on the Straight Creek Fault;[104] these may reflect the last movement of Siletzia relative to North America. On a broader scale, there was a change in absolute direction of the Pacific plate[105] (marked by the end of the bend in the Hawaiian-Emperor seamount chain), and a change in the convergence of the Kula plate with the North American plate.[106]
As subduction waned so did the force that had clamped Siletzia against the continent, and the tectonic regime shifted from compressional to extensional.[107] Deposition of sand from the then proximal Idaho Batholith into the Tyee Formation in southern Oregon may have continued as late as 46.5 Ma,[108] but was interrupted when Siletzia rifted from the continent and began rotating away.[109] What initiated rifting is unknown. Wells et al. (1984, p. 290) suggested that as the continent overrode the Yellowstone hotspot, the upwelling plume tore away a previously accreted terrane. Babcock et al. (1992) suggested a change in the rate at which the plates were converging, or the "kinematic effects" (such as a slab window) from the passage of the Kula-Farallon ridge (or Resurrection-Farallon ridge).[110]
See also
Notes
- ↑ Snavely, MacLeod & Wagner 1968, p. 454; Phillips, Walsh & Hagen 1989, p. 209; Brandon & Vance 1992, p. 571; Trehu et al. 1994, p. 237.
- ↑ Silberling et al. 1987. The portion of Siletzia under Oregon and southeastern Washington, leaving out the Olympic Peninsula and Vancouver Island, has also been called the Willamette Plate. Magill et al. 1982, p. 3771, and see fig. 11, p. 3772.
- ↑ Brandon & Vance 1992, p. 571.
- ↑ Brandon & Vance 1992, p. 571.
- ↑ Phillips, Walsh & Hagen 1989, pp. 200, 205; Cady 1975, p. 573.
- ↑ Phillips, Walsh & Hagen 1989. Authors have differed as to which formations are Siletzian. For a recent categorization see McCrory & Wilson 2013b, Table 1.
- ↑ Bromley 2011, p. 9; McCrory & Wilson 2013b, para. 2.
- ↑ Sharp & Clague 2006, p. 1283.
- ↑ Gao 2011, pp. 44, 48.
- ↑ Wells et al. 1984, Fig. 1; Trehu et al. 1994, note 9; McCrory & Wilson 2013b, §2.1, Fig. 1, and Table 1.
- ↑ Chan, Tepper & Nelson 2012, p. 1324; Rarey 1985, p. 87.
- ↑ Arnold 1906.
- ↑ Babcock, Suczek & Engebretson 1994, p. 144.
- ↑ Henriksen 1956, pp. 22, 31.
- ↑ Weaver 1939.
- ↑ Originally named the "Siletz River Volcanic Series" by Snavely & Baldwin 1948, renamed by Snavely, MacLeod & Wagner 1968, p. 454.
- ↑ Snavely, MacLeod & Wagner 1968; Baldwin 1974; Wells et al. 1984; Baldwin & Perttu 1989; Wells et al. 2000.
- ↑ Irving 1979, p. 672.
- ↑ Wells, Weaver & Blakely 1998, p. 760.
- ↑ Wells et al. 2000, p. 15.
- ↑ Massey 1986, p. 602.
- ↑ Brandon & Vance 1992, p. 571.
- ↑ The Leech River Fault/CRBF has also been aligned with possible faults in Discovery Bay and Puget Sound — see Puget Sound faults — but the evidence is rather against these possibilities. E.g., see Babcock et al. 1992, p. 6809 and Babcock, Suczek & Engebretson 1994, p. 149.
- ↑ Finn 1990, p. 19,537.
- ↑ Stanley, Finn & Plesha 1987, p. 10,179.
- ↑ Miller et al. 1997, p. 17,869.
- ↑ Stanley, Finn & Plesha 1987, p. 10,186; Stanley et al. 1996, pp. 4,16.
- ↑ Blakely 1994, p. 2771.
- ↑ Blakely 1994, p. 2759; Gao et al. 2011, pp. 206, 208, 210.
- ↑ Wells et al. 2000, p. 12, and figure 2, p. 31.
- ↑ Snavely & Wells 1996, pp. 162, 171 and Fig. 64; Goldfinger et al. 1997, Fig. 2.
- ↑ Fleming & Tréhu 1999, pp. 20,442, 20,432; Snavely & Wells 1996, pp. 172–173; Davis & Plafker 1986.
- ↑ Goldfinger et al. 1997, p. 8228. Parsons et al. (1999) used seismic data to build a three-dimensional image of Siletzia beneath Washington, including the inferred western boundary.
- ↑ Beck & Engebretson 1982, pp. 3757-56.
- ↑ Cady 1975; Warnock, Burmester & Engebretson 1993, pp. 11,735–36.
- ↑ Trehu et al. 1994; McCrory & Wilson 2013a. McCrory & Wilson (2013b, para. 7) say 27±5 km.
- ↑ Graindorge et al. 2003, §9.2; McCrory & Wilson 2013a, slides 15 and 17.
- ↑ Cady 1975, p. 573 and following sources.
- ↑ Snavely, MacLeod & Wagner 1968, p. 480. A more detailed description of the Siletz River Volcanics can be found in Snavely, Wagner & MacLeod 1965, and of the Crescent Formation in Lyttle & Clarke 1975.
- ↑ Cady 1975, p. 579.
- ↑ Snavely & Wells 1996, p. 164.
- ↑ Heller & Ryberg 1983, p. 380; Heller, Tabor & Suczek 1987, p. 1662; Grommé et al. 1986, p. 14,090; Goldfinger 1990, p. 12.
- ↑ Heller et al. 1985, p. 779.
- ↑ Duncan 1982.
- ↑ Babcock et al. 1992, p. 6815. Variations in geochemical alteration may also have skewed the results. Duncan 1982, p. 10,828; Magill, Cox & Duncan 1981, p. 2956.
- ↑ Pyle et al. 2009 (abstract); Wells et al. 2010 (abstract).
- ↑ Trehu et al. 1994, Fig. 2.
- ↑ Weaver 1939.
- ↑ Quoted in Henriksen 1956, p. 111.
- ↑ Snavely, MacLeod & Wagner 1968, p. 456.
- ↑ Babcock et al. 1992, p. 6813.
- ↑ Wells et al. 2010 (abstract).
- ↑ Beck & Plumley 1980, p. 573; Bates, Beck & Burmester 1981, p. 188.
- ↑ Beck & Plumley 1980, p. 573; Magill, Cox & Duncan 1981, p. 2958. Other possible rotation mechanisms are discussed by Globerman, Beck & Duncan 1982, p. 1156. See also Wells & Heller 1988.
- ↑ Second model of Simpson & Cox (1977), as elaborated by Hammond (1979). Several objections to a northern pivot were made by Magill, Cox & Duncan (1981), who favored an initial phase of rotation with a southern pivot (p. 2960). Some apparent palinspastic conflicts involving the Clarno Formation of north-central Oregon (Simpson & Cox 1977, p. 588) appear to have been resolved by Grommé et al. (1986). A major problem for a southern pivot is that it implies rotation during accretion, while most studies indicate that most or all of the rotation occurred after the presumed accretion (Heller et al. 1985, p. 779).
- ↑ Cady 1975, p. 579. See also Babcock, Suczek & Engebretson 1994, pp. 141, 144 and McCrory & Wilson 2013b, §2.1.2.
- ↑ Heller et al. 1985, pp. 770, 773, 779; Dumitru et al. 2013
- ↑ Wells & Simpson 2001.
- ↑ Zandt & Humphreys 2009; Wells & McCaffrey 2013.
- ↑ McCrory & Wilson 2013b, paragraphs 5, 50, 54, 63-66.
- ↑ Magill & Cox 1981; Globerman, Beck & Duncan 1982, p. 1155; Wells et al. 1984, p. 280.
- ↑ Blakely et al. 2002.
- ↑ Beck & Engebretson 1982.
- ↑ Johnston & Acton 2003.
- ↑ Prothero, Draus & Burns 2009.
- ↑ Bromley 2011, p. 9.
- ↑ McCrory & Wilson 2013b, para. 2.
- ↑ Brandon & Vance (1992, p. 571) call these the seamount interpretation and the marginal basin interpretation. Chan, Tepper & Nelson (2012, p. 1324) count only three general models, restricting the first to hotspot volcanism on a spreading ridge, and counting slab windows as a third model.
- ↑ Some early models had Siletzia rotating into the continent about a southern pivot, accretion therefore being the culmination of rotation. The southern pivot seems to be largely abandoned, in part because various studies (e.g.: Heller & Ryberg 1983, p. 383; Wells et al. 1984, p. 280; Heller et al. 1985, p. 779) show most of the rotation was post-accretion. These classes of models have been classified as either "accreted" or "rifted," but this is inaccurate as inshore formation can still involve accretion, and all offshore accretion models using a northern pivot imply rifting.
- ↑ Babcock et al. 1992, p. 6813.
- ↑ Bromley 2011, p. 9.
- ↑ Babcock et al. (1992, fig. 10) show the uncertainly in the position of the K-F ridge at 65 Ma as anywhere from Mexico to the Queen Charlotte Islands. See also figure 1 in Haeussler et al. 2003, showing the K-F ridge alternately near Washington, or near Anchorage.
- ↑ Cady 1975, p. 579.
- ↑ Duncan 1982, p. 10,828.
- ↑ Duncan 1982, pp. 10,828, 10,830.
- ↑ Chan, Tepper & Nelson 2012, p. 1324. And substantially younger, at 42 to 37 Ma.
- ↑ Pyle et al. 2009.
- ↑ Wells et al. 1984, p. 280.
- ↑ Wilson & McCrory 2010. See also McCrory & Wilson 2013b.
- ↑ Wells et al. 1984, p. 289.
- ↑ Lonsdale 1988, p. 752.
- ↑ Wells et al. 1984, pp. 289–290.
- ↑ Babcock et al. 1992, p. 6813.
- ↑ Thorkelson 1996.
- ↑ Michaud et al. 2002; Thorkelson 1996, p. 48.
- ↑ Madsen et al. 2006, p. 31. Their model has the northern part of the Resurrection plate separating at about 47 Ma to form the Eshamy plate.
- ↑ Babcock et al. 1992, p. 6819. See also Christiansen, Foulger & Evans 2002.
- ↑ See figure 1 in Haeussler et al. 2003, p. 868.
- ↑ Haeussler et al. 2003, p. 872.
- ↑ Davis & Plafker 1986. See also Cowan 2003, p. 472.
- ↑ Cowan 2003, pp. 465–471 and figure 4.
- ↑ Simpson & Cox 1977, p. 588, figure 5; see also Hamilton 1969, fig. 4. This would be the Challis subduction zone, but there is some question about it. See Babcock et al. 1992, p. 6817; Brandon & Vance 1992, p. 570; Schmandt & Humphreys 2010, p. 7.
- ↑ Heller & Ryberg 1983, p. 383; Phillips, Walsh & Hagen 1989, p. 199. Some early studies (e.g., Duncan 1982) dated accretion as late as 42 Ma. A recent study (Schmandt & Humphreys 2011) suggests it may have been as early as 55 Ma.
- ↑ Wells et al. 1984, pp. 275, 290; Heller, Tabor & Suczek 1987, p. 1652; Babcock et al. 1992, p. 5814; Gao et al. 2011, pp. 1, 43, 58.
- ↑ Haeussler et al. 2003, p. 872.
- ↑ Vance & Miller 1994.
- ↑ Gao 2011, p. 9; Schmandt & Humphreys 2011, p. 177.
- ↑ Moye et al. 1988; Babcock et al. 1992, p. 6817; du Bray & John 2011, p. 1122.
- ↑ Dickinson 1976, p. 1283; Oxford 2006, p. 12; Gao et al. 2011, p. 203. How this happened does not seem to be detailed anywhere, but figure 5 of Simpson & Cox (1977, p. 587) suggests that the new subduction zone may have simply unzipped from the old zone, starting from the south.
- ↑ Chan, Tepper & Nelson 2012, p. 1324.
- ↑ Babcock et al. 1992, p. 6817.
- ↑ Babcock et al. 1992, p. 6813.
- ↑ Clowes et al. 1987, p. 33.
- ↑ Vance & Miller 1994.
- ↑ Wells et al. 1984, p. 277.
- ↑ Lonsdale 1988, p. 33.
- ↑ Heller, Tabor & Suczek 1987, p. 1652.
- ↑ Dumitru et al. 2013, p. 188.
- ↑ As explained earlier, it appears the rotation was about a northern pole.
- ↑ Babcock et al. 1992, pp. 6799, 6819, 6813.
References
- Arnold, R. (24 September 1906), "Geological reconnaissance of the coast of the Olympic Mountains.", GSA Bulletin, 17: 451–468, doi:10.1130/GSAB-17-451.
- Babcock, R. S.; Burmester, R. F.; Engebretson, D. C.; Warnock, A.; Clark, K. P. (1992), "A Rifted Margin Origin for the Crescent Basalt and Related Rocks in the Northern Coast Range Volcanic Province, Washington and British Columbia", Journal of Geophysical Research, 97 (B5): 6,799–6,821, Bibcode:1992JGR....97.6799B, doi:10.1029/91jb02926.
- Babcock, R. S.; Suczek, C. A.; Engebretson, D. C. (1994), "The Crescent "Terrane", Olympic Peninsula and Southern Vancouver Island", in Lasmanis, Raymond; Cheney, Eric S., Regional Geology of Washington State (PDF), DGER Bulletin, 80, pp. 141–157.
- Baldwin, E. M. (1974), "Eocene stratigraphy of southwestern Oregon", Oregon DOGAMI Bulletin, 83, 1 plate, scale 1:250,000, with 40 p. text.
- Baldwin, E. M.; Perttu, R. K. (January 1989), "Eocene unconformities in the Camas Valley quadrangle, Oregon" (PDF), Oregon Geology, 51 (1): 3–8.
- Bates, R. G.; Beck, M. E.; Burmester, R. F. (April 1981), "Tectonic rotations in the Cascade Range of southern Washington", Geology, 9 (4): 184–189, doi:10.1130/0091-7613(1981)9<184:TRITCR>2.0.CO;2.
- Beck, M. E.; Engebretson, D. C. (10 May 1982), "Paleomagnetism of small basalt exposures in the west Puget Sound area, Washington, and speculations on the accretionary origin of the Olympic Mountains", Journal of Geophysical Research, 87 (B5): 3755–3760, Bibcode:1982JGR....87.3755B, doi:10.1029/JB087iB05p03755.
- Beck, M. E.; Plumley, P. W. (December 1980), "Paleomagnetism of intrusive rocks in the Coast Range of Oregon: Microplate rotations in middle Tertiary time", Geology, 8 (12): 573–577, doi:10.1130/0091-7613(1980)8<573:POIRIT>2.0.CO;2.
- Blakely, R. J. (10 November 1994), "Volcanism, Isostatic Residual Gravity, and Regional Tectonic Setting of the Cascade Volcanic Province", Journal of Geophysical Research, 90 (B2): 2757–2773, Bibcode:1990JGR....9519439B, doi:10.1029/jb095ib12p19439.
- Blakely, R. J.; Wells, R. E.; Weaver, C. S.; Meagher, K. L.; Ludwin, R. (May 2002), "The bump and grind of Cascadia forearc blocks: evidence from gravity and magnetic anomalies [abstract]", GSA Abstracts with Programs, 34 (5).
- Brandon, M. T.; Vance, J. A. (October 1992), "Tectonic evolution of the Cenozoic Olympic subduction complex, Washington State, as deduced from fission track ages for detrital zircons" (PDF), AJS, 293: 565–636, doi:10.2475/ajs.292.8.565.
- du Bray, E. A.; John, D. A. (October 2011), "Petrologic, tectonic, and metallogenic evolution of the ancestral Cascades magmatic arc, Washington, Oregon, and Northern California", Geosphere, 7 (5): 1102–1133, doi:10.1130/GES00669.1.
- Breitsprecher, K.; Thorkelson, D. J.; Groome, W. G.; Dostal, J. (April 2003), "Geochemical confirmation of the Kula-Farallon slab window beneath the Pacific Northwest in Eocene time" (PDF), GSA Bulletin, 31 (4): 351–354, doi:10.1130/0091-7613(2003)031<0351:gcotkf>2.0.co;2.
- Bromley, S. A. (June 2011), Evolution and Inheritance of Cascadia Sub-arc Mantle Reservoirs [masters thesis], Oregon State University.
- Cady, W. M. (September–October 1975), "Tectonic setting of the Tertiary volcanic rocks of the Olympic Peninsula, Washington" (PDF), USGS Journal of Research, 3 (5): 573–582.
- Chan, C. F.; Tepper, J. H.; Nelson, B. K. (July–August 2012), "Petrology of the Grays River volcanics, southwest Washington: Plume-influenced slab window magmatism in the Cascadia forearc", GSA Bulletin, 124 (7-8): 1324–1338, doi:10.1130/B30576.1.
- Christiansen, R. L.; Foulger, G. R.; Evans, J. R. (October 2002), "Upper-mantle origin of the Yellowstone hotspot" (PDF), GSA Bulletin, 114 (10): 1245–1256, doi:10.1130/0016-7606(2002)114<1245:UMOOTY>2.0.CO;2.
- Clowes, R. M.; Brandon, M. T.; Green, A. G.; Yorath, C. J.; Brown, A. S.; Kanasewich, E. R.; Spencer, C. (January 1987), "LITHOPROBE— southern Vancouver Island: Cenozoic subduction complex imaged by deep seismic reflections" (PDF), Canadian Journal of Earth Sciences, 24 (1): 31–51, Bibcode:1987CaJES..24...31C, doi:10.1139/e87-004.
- Cowan, D. S. (25 August 2003), "Revisiting the Baranof-Leech River hypothesis for early Tertiary coastwise transport of the Chugach-Prince William Terrane", Earth and Planetary Science Letters, 213 (3-4): 463–475, Bibcode:2003E&PSL.213..463C, doi:10.1016/S0012-821X(03)00300-5.
- Davis, A. S.; Plafker, G. (November 1986), "Eocene basalts from the Yakutat Terrane; evidence for the origin of an accreting terrane in southern Alaska", Geology, 14: 963–966, doi:10.1130/0091-7613(1986)14<963:EBFTYT>2.0.CO;2.
- Dickinson, W. R. (September 1976), "Sedimentary basins developed during evolution of Mesozoic-Cenozoic arc-trench system in western North America", Canadian Journal of Earth Sciences, 13 (9): 1268–1287, doi:10.1139/e76-129.
- Dickinson, W. R.; Snyder, W. S. (1979), "Geometry of subducted slabs related to San Andreas transform", Journal of Geology, 87: 609–627, doi:10.1086/6284.
- Dumitru, T. A.; Ernst, W. G.; Wright, J. E.; Wooden, J. L.; Wells, R. E.; Farmer, L. P.; Kent, A. J. R.; Graham, S. A. (February 2013), "Eocene extension in Idaho generated massive sediment floods into the Franciscan trench and into the Tyee, Great Valley, and Green River basins", Geology, 41 (2): 187–190, doi:10.1130/G33746.1.
- Duncan, R. A. (10 December 1982), "A captured island chain in the coast range of Oregon and Washington" (PDF), Journal of Geophysical Research, 87 (B13): 10,827–10,837, Bibcode:1982JGR....8710827D, doi:10.1029/jb087ib13p10827.
- Finn, C. (10 November 1990), "Geophysical constraints on Washington convergent margin structure", Journal of Geophysical Research, 95 (B12): 19,533–19,546, Bibcode:1990JGR....9519533F, doi:10.1029/jb095ib12p19533.
- Fleming, S. W.; Tréhu, A. M. (10 September 1999), "Crustal structure beneath the central Oregon convergent margin from potential-field modeling: Evidence for a buried basement ridge in local contact with a seaward dipping backstop" (PDF), Journal of Geophysical Research, 104 (B9): 20,431–20,447, Bibcode:1999JGR...10420431F, doi:10.1029/1999jb900159.
- Gao, H. (March 2011), The seismic structures of the U.S. Pacific Northwest and the scaling and recurrence patterns of the slow slip events [dissertation] (PDF), University of Oregon.
- Gao, H.; Humphreys, E. D.; Yao, H.; van der Hilst, R. D. (2011), "Crust and lithosphere structure of the northwestern U.S. with ambient noise tomography: Terrane accretion and Cascade arc development", Earth and Planetary Science Letters, 304: 202–211, Bibcode:2011E&PSL.304..202G, doi:10.1016/j.epsl.2011.01.033. Available on Google Books.
- Globerman, B. R.; Beck, M. E.; Duncan, R. A. (November 1982), "Paleomagnetism and tectonic significance of Eocene basalts from the Black Hills, Washington Coast Range", GSA Bulletin, 91 (11): 1151–1159, doi:10.1130/0016-7606(1982)93<1151:PATSOE>2.0.CO;2.
- Goldfinger, C. (May 1990), Evolution of the Corvallis Fault and Implications for the Oregon Coast Range [masters thesis], Oregon State University.
- Goldfinger, C.; Kulm, L. D.; Yeats, R. S.; McNeill, L.; Hummon, C. (10 April 1997), "Oblique strike-slip faulting of the central Cascadia submarine forearc" (PDF), Journal of Geophysical Research, 102 (B4): 8217–8243, Bibcode:1997JGR...102.8217G, doi:10.1029/96jb02655.
- Graindorge, D.; Spence, G.; Charvis, P.; Collot, J. Y.; Hyndman, R.; Trehu, A. M. (October 2003), "Crustal structure beneath the Strait of Juan de Fuca and southern Vancouver Island from seismic and gravity analyses" (PDF), Journal of Geophysical Research: Solid Earth, 108 (B10), Bibcode:2003JGRB..108.2484G, doi:10.1029/2002JB001823.
- Grommé, C. S.; Beck, M. E.; Wells, R. E.; Engebretson, D. C. (10 December 1986), "Paleomagnetism of the Tertiary Clarno Formation and its Significance for the Tectonic History of the Pacific Northwest", Journal of Geophysical Research, 91 (B14): 14,089–14,104, Bibcode:1986JGR....9114089G, doi:10.1029/JB091iB14p14089.
- Haeussler, P. J.; Bradley, D. C.; Wells, R. E.; Miller, M. L. (July 2003), "Life and death of the Resurrection plate: Evidence for its existence and subduction in the northeastern Pacific in Paleocene-Eocene time" (PDF), GSA Bulletin, 115 (7): 867–880, doi:10.1130/0016-7606(2003)115<0867:LADOTR>2.0.CO;2.
- Hamilton, W. (December 1969), "California and the underflow of the Pacific mantle", GSA Bulletin, 80 (12): 2409–2430, doi:10.1130/0016-7606(1969)80[2409:MCATUO]2.0.CO;2.
- Hammond, P. E. (14 March 1979), "A tectonic model for evolution of the Cascade Range", in Armentrout, J. M.; Cole, M. R.; TerBest, Jr., H., The Cenozoic paleogeography of the Western United States, Society of Economic Paleontologists and Mineralologists, pp. 219–237.
- Heller, P. L.; Peterman, Z. E.; O'Neil, J. R.; Shafiquallah, M. (June 1985), "Isotopic provenance of sandstones from the Eocene Tyee Formation, Oregon Coast Range", GSA Bulletin, 96 (6): 770–780, doi:10.1130/0016-7606(1985)96<770:IPOSFT>2.0.CO;2.
- Heller, P. L.; Ryberg, P. T. (July 1983), "Sedimentary record of subduction to forearec transition in the rotated Eocene basin of western Oregon", Geology, 11: 380–383, doi:10.1130/0091-7613(1983)11<380:SROSTF>2.0.CO;2.
- Heller, P. L.; Tabor, R. W.; Suczek, C. A. (August 1987), "Paleogeographic evolution of the U.S. Pacific Northwest during Paleogene time" (PDF), Canadian Journal of Earth Sciences, 24 (8): 1652–1667, doi:10.1139/e87-159.
- Henriksen, D. A. (1956), "Eocene stratigraphy of the lower Cowlitz River–eastern Willapa Hills area, southwestern Washington" (PDF), Washington Division of Mines and Geology Bulletin, 43.
- Irving, E. (March 1979), "Paleopoles and paleolatitudes of North America and speculations about displaced terranes", Canadian Journal of Earth Sciences, 16 (3): 669–694, doi:10.1139/e79-065.
- Johnston, S. T.; Acton, S. (24 April 2003), "The Eocene Southern Vancouver Island Orocline — a response to seamount accretion and the cause of fold-and-thrust belt and extensional basin formation" (PDF), Tectonophysics, 365 (104): 165–183, Bibcode:2003Tectp.365..165J, doi:10.1016/S0040-1951(03)00021-0.
- Lonsdale, P. (May 1988), "Paleogene history of the Kula plate: offshore evidence and onshore implications", GSA Bulletin, 100 (5): 733–754, doi:10.1130/0016-7606(1988)100<0733:PHOTKP>2.3.CO;2.
- Lyttle, N. A.; Clarke, D. B. (March 1975), "New analyses of Eocene basalts from the Olympic Peninsula, Washington", GSA Bulletin, 86 (3): 421–427, doi:10.1130/0016-7606(1975)86<421:NAOEBF>2.0.CO;2.
- Madsen, J. K.; Thorkelson, D. J.; Friedman, R. M.; Marshall, D. D. (February 2006), "Cenozoic to Recent plate configurations in the Pacific Basin: Ridge subduction and slab window magmatism in western North America" (PDF), Geosphere, 2 (1): 11–34, doi:10.1130/GES00020.1.
- Magill, J. R.; Cox, A. V. (March 1981), "Post-Oligocene tectonic rotation of the Oregon Western Cascade Range and the Klamath Mountains", Geology, 9: 127–131, doi:10.1130/0091-7613(1981)9<127:PTROTO>2.0.CO;2.
- Magill, J. R.; Cox, A. V.; Duncan, R. (10 April 1981), "Tillamook Volcanic Series: Further evidence for tectonic rotation of the Oregon Coast Ranges" (PDF), Journal of Geophysical Research, 86 (B4): 2953–2970, Bibcode:1981JGR....86.2953M, doi:10.1029/JB086iB04p02953.
- Magill, J. R.; Wells, R. E.; Simpson, R. W.; Cox, A. V. (10 May 1982), "Post-12 m.y. rotation of southwest Washington", Journal of Geophysical Research, 87 (B5): 3761–3776, Bibcode:1982JGR....87.3761M, doi:10.1029/jb087ib05p03761.
- Massey, N. W. D. (June 1986), "Metchosin Igneous Complex, southern Vancouver Island: Ophiolite stratigraphy developed in an emergent island setting", Geology, 14 (7): 602–605, doi:10.1130/0091-7613(1986)14<602:MICSVI>2.0.CO;2.
- McCrory, P.; Wilson, D. S. (March 2013a), Siletz‐Crescent Forearc Terrane Revisited » Does a New Kinematic Model Solve an Old Puzzle?, Menlo Park (Calif.): United States Geological Survey.
- McCrory, P.; Wilson, D. S. (June 2013b), "A kinematic model for the formation of the Siletz-Crescent forearc terrane by capture of coherent fragments of the Farallon and Resurrection plates.", Tectonics, 32 (3): 718–736, doi:10.1002/tect.20045
- McWilliams, R. G. (September 1980), "Eocene correlations in western Oregon-Washington" (PDF), Oregon Geology, 42 (9): 151–158.
- Michaud, F.; Bourgois, J.; Royer, J.; Dyment, J.; Sichler, B.; Bandy, W.; Mortera, C.; Calmus, T.; Vieyra, M.; Sosson, M.; Pontoise, B.; Bigot-Cormier, F.; Diaz, O.; Hurtado, A.; Pardo, G.; Trouillard-Perrot, C. (Fall 2002), "The FAMEX Cruise off Baja California (March/April 2002): Preliminary Results [abstract #T52A-1186]", American Geophysical Union, Fall Meeting, Bibcode:2002agufm.t52a1186m.
- Miller, K. C.; Keller, G. R.; Gridley, J. M.; Luetgert, J. H.; Mooney, W. D.; Thybo, H. (10 August 1997), "Crustal structure along the west flank of the Cascades, western Washington", Journal of Geophysical Research, 102 (B8): 17,857–17,873, Bibcode:1997JGR...10217857M, doi:10.1029/97jb00882.
- Miner, A. M. (June 2002), Eocene tectonics and active deformation in Cascadia [masters thesis], Central Washington University.
- Moye, F. J.; Hackett, W. R.; Blakely, J. D.; Snider, L. G. (1988), "Regional geologic setting and volcanic stratigraphy of the Challis volcanic field, central Idaho", in P. K., Link; W. R., Hackett, Guidebook to the Geology of Central and Southern Idaho (PDF), Idaho Geological Survey Bulletin, 27, pp. 87–97.
- Oxford, J. (October 2006), Early Oligocene Intrusions in the Central Coast Range of Oregon: Petrography, Geochemistry, Geochronology and Implications for the Tertiary Magmatic Evolution of the Cascadia Forearc [masters thesis], Oregon State University.
- Parsons, T.; Wells, R. E.; Fisher, M. A.; Flueh, E.; ten Brink, U. S. (10 August 1999), "Three-dimensional velocity structure of Siletzia and other accreted terranes in the Cascadia forearc of Washington" (PDF), Journal of Geophysical Research, 104 (B8): 18,015–18,039, Bibcode:1999JGR...10418015P, doi:10.1029/1999jb900106.
- Phillips, W. M.; Walsh, T. J.; Hagen, R. A. (December 1989), "Eocene transition from oceanic to arc volcanism, southwest Washington", in Muffler, L. J.; Weaver, C. S.; Blackwell, D. D., Proceedings of workshop XLIV: Geological, geophysical, and tectonic setting of the Cascade Range (PDF), U.S. Geological Survey, Open-File Report 89-176, pp. 199–256.
- Prothero, D. R.; Draus, E.; Burns, B. (2009), "Magnetostratigraphy and Tectonic Rotation of the Eocene-Oligocene Makah and Hoko River Formations, Northwest Washington, USA" (PDF), International Journal of Geophysics, 2009: 1–15, doi:10.1155/2009/930612.
- Pyle, D. G.; Duncan, R.; Wells, R. E.; Graham, D. W.; Harrison, B.; Hanan, B. (October 2009), "Siletzia: an oceanic large igneous province in the Pacific Northwest [abstract]", GSA Abstracts with Programs, 41 (7): 369.
- Rarey, P. J. (1985), Geology of the Hamlet-North Fork of the Nehalem River Area, Southern Clatsop and Northernmost Tillamook Counties, Northwest Oregon [masters thesis], Oregon State University.
- Schmandt, B.; Humphreys, E. (September 2010), "Complex subduction and small-scale convection revealed by body-wave tomography of the western United States upper mantle" (PDF), Earth and Planetary Science Letters, 297 (3-4): 435–445, Bibcode:2010E&PSL.297..435S, doi:10.1016/j.epsl.2010.06.047.
- Schmandt, B.; Humphreys, E. (February 2011), "Seismically imaged relict slab from the 55 Ma Siletzia accretion to the northwest United States" (PDF), Geology, 39 (2): 175–178, doi:10.1130/G31558.1.
- Sharp, W. D.; Clague, D. A. (September 2006), "50-Ma Initiation of Hawaiian-Emperor Bend Records Major Change in Pacific Plate Motion", Science, 313: 1281–1284, doi:10.1126/science.1128489, PMID 16946069.
- Silberling, N. J.; Jones, D. L.; Blake, Jr., M. C.; Howell, D. G. (1987), "Lithotectonic terrane map of the western conterminous-United States", U.S. Geological Survey, Miscellaneous Field Studies Map MF-1874-C, 1 sheet, scale 1:2,500,00, 20 p. text.
- Simpson, R. W.; Cox, A. (October 1977), "Paleomagnetic evidence for tectonic rotation of the Oregon Coast Range", Geology, 5: 585–589, doi:10.1130/0091-7613(1977)5<585:PEFTRO>2.0.CO;2.
- Snavely, P. D.; Baldwin, E. M. (1948), "Siletz River Volcanic Series, northwestern Oregon", AAPG Bulletin, 32: 805–812, doi:10.1306/3d933b78-16b1-11d7-8645000102c1865d.
- Snavely, P. D.; MacLeod, N. S.; Wagner, H. C. (June 1968), "Tholeiitic and alkalic basalts of the Eocene Siletz River Volcanics, Oregon Coast Range", American Journal of Science, 266: 454–481, doi:10.2475/ajs.266.6.454.
- Snavely, P. D.; Wagner, H. C.; MacLeod, N. S. (June 1965), "Preliminary data on compositional variations of Tertiary volcanic rocks in the central part of the Oregon Coast Range" (PDF), The Ore Bin, 27 (6): 101–117.
- Snavely, P. D.; Wells, R. E. (1991), "Cenozoic evolution of the continental margin of Oregon and Washington" (PDF), U.S. Geological Survey, Open-File Report 91-441-B.
- Snavely, P. D.; Wells, R. E. (1996), "Cenozoic evolution of the continental margin of Oregon and Washington", in Rogers, A. M.; Walsh, T. J.; Kockelman, W. J.; Priest, G. R., Assessing Earthquake Hazards and Reducing Risk in the Pacific Northwest, Vol. 1, Professional Paper 1560, vol. 1, U.S. Geological Survey, pp. 161–182.
- Stanley, W. D.; Finn, C.; Plesha, J. L. (September 1987), "Tectonics and Conductivity Structures in the Southern Washington Cascades", Journal of Geophysical Research, 92 (B10): 10,179–10,193, Bibcode:1987JGR....9210179S, doi:10.1029/jb092ib10p10179.
- Stanley, W. D.; Johnson, S. Y.; Qamar, A. I.; Weaver, C. S.; Williams, J. M. (February 1996), "Tectonics and Seismicity of the Southern Washington Cascade Range" (PDF), Bulletin of the Seismological Society of America, 86 (1A): 1–18.
- Thorkelson, D. J. (20 April 1996), "Subduction of diverging plates and the principles of slab window formation" (PDF), Tectonophysics, 255 (1-2): 47–63, doi:10.1016/0040-1951(95)00106-9.
- Thorkelson, D. J.; Taylor, R. P. (September 1989), "Cordilleran slab windows" (PDF), Geology, 17: 833–836, doi:10.1130/0091-7613(1989)017<0833:csw>2.3.co;2.
- Trehu, A. M.; Asudeh, I.; Brocher, T. M.; Luetgert, J. H.; Mooney, W. D.; Nabelek, J. L.; Nakamura, Y. (14 October 1994), "Crustal architecture of the Cascadia forearc" (PDF), Science, 266: 237–243, doi:10.1126/science.266.5183.237.
- Vance, J. A.; Miller, R. B. (1994), "Another look at the Fraser River-Straight Creek Fault (FRSCF) [GSA Abstract 5451]", in R., Lasmanis; E. S., Cheney, Regional Geology of Washington State (PDF), Bulletin, 80, Washington DGER, p. 88.
- Warnock, A. C.; Burmester, R. F.; Engebretson, D. C. (10 July 1993), "Paleomagnetism and tectonics of the Crescent Formation, northern Olympic Mountains, Washington", Journal of Geophysical Research, 98 (B7): 11,729–41, Bibcode:1993JGR....9811729W, doi:10.1029/93jb00709.
- Weaver, C. E. (1 December 1939), "Metchosin volcanic rock in Oregon and Washington [abstract]", GSA Bulletin, 50 (12, part 2): 1961, doi:10.1130/GSAB-50-1945.
- Wells, R. E.; Bukry, D.; Wooden, J. L.; Friedman, R. M.; Haeussler, P. J. (Fall 2010), Magmatic and kinematic history of Siletzia, a Paleocene-Eocene accreted oceanic terrane in the Oregon Coast Range [abstract #T12C-06], American Geophysical Union, Bibcode:2010AGUFM.T12C..06W.
- Wells, R. E.; Engebretson, D. C.; Snavely, P. D.; Coe, R. S. (April 1984), "Cenozoic Plate Motions and the Volcano-Tectonic Evolution of Western Oregon and Washington" (PDF), Tectonics, 3 (2): 275–294, Bibcode:1984Tecto...3..275W, doi:10.1029/TC003i002p00275.
- Wells, R. E.; Heller, P. L. (March 1988), "The relative contribution of accretion, shear, and extension to Cenozoic tectonic rotation in the Pacific Northwest", GSA Bulletin, 100 (3): 325–338, doi:10.1130/0016-7606(1988)100<0325:TRCOAS>2.3.CO;2.
- Wells, R. E.; Jayko, A. S.; Niem, A. R.; Black, G.; Wiley, T.; Baldwin, E.; Molenaar, K. M.; Wheeler, K. L.; DuRoss, C. B.; Givler, R. W. (2000), "Geologic Map and Database of the Roseburg 30 x 60' Quadrangle, Douglas and Coos Counties, Oregon" (PDF), U.S. Geological Survey, Open-File Report 00-376.
- Wells, R. E.; McCaffrey, R. (September 2013), "Steady rotation of the Cascade arc", Geology, 41 (9): 1027–1030, doi:10.1130/G34514.1.
- Wells, R. E.; Simpson, R. W. (2001), "Northward migration of the Cascadia forearc in the northwestern U.S. and implications for subduction deformation" (PDF), Earth, Planets and Space, 53: 275–283, doi:10.1186/bf03352384.
- Wells, R. E.; Weaver, C. S.; Blakely, R. J. (August 1998), "Forearc migration in Cascadia and its neotectonic significance" (PDF), Geology, 26 (8): 759–762, doi:10.1130/0091-7613(1998)026<0759:famica>2.3.co;2.
- Wilson, D. S.; McCrory, P. A. (Fall 2010), A kinematic model for the formation of the Siletz terrane by capture of coherent fragments of the Farallon and Resurrection plates [abstract #T13C-2210], American Geophysical Union, Bibcode:2010AGUFM.T13C2210W.
- Zandt, G.; Humphreys, G. (April 2009), "Toroidal mantle flow through the western U.S. slab window" (PDF), Geology, 36 (4): 295–298, doi:10.1130/G24611A.1.