Properties of metals, metalloids and nonmetals
.png)
metals in most of the left and centre;
metalloids in a narrow diagonal band;
nonmetals in the right, and hydrogen
The chemical elements can be broadly divided into metals, metalloids and nonmetals according to their shared physical and chemical properties. All metals have a shiny appearance (at least when freshly polished); are good conductors of heat and electricity; form alloys with other metals; and have at least one basic oxide. Metalloids are metallic-looking brittle solids that are either semiconductors or exist in semiconducting forms, and have amphoteric or weakly acidic oxides. Typical nonmetals have a dull, coloured or colourless appearance; are brittle when solid; are poor conductors of heat and electricity; and have acidic oxides. Most or some elements in each category share a range of other properties; a few elements have properties that are either anomalous given their category, or otherwise extraordinary.
Shared properties
Metals
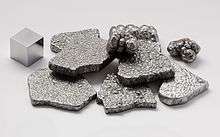
Metals appear lustrous (beneath any patina); form mixtures (alloys) when combined with other metals; tend to lose or share electrons when they react with other substances; and each forms at least one predominantly basic oxide.
Most metals are silvery looking, high density, relatively soft and easily deformed solids with good electrical and thermal conductivity, closely packed structures, low ionisation energies and electronegativities, and are found naturally in combined states.
Some metals appear coloured (Cu, Cs, Au), have low densities (e.g. Be, Al) or very high melting points, are liquids at or near room temperature, are brittle (e.g. Os, Bi), not easily machined (e.g. Ti, Re), or are noble (hard to oxidise) or have nonmetallic structures (Mn and Ga are structurally analogous to, respectively, white P and I).
Metals comprise the large majority of the elements, and can be subdivided into several different categories. From left to right in the periodic table, these categories include the highly reactive alkali metals; the less reactive alkaline earth metals, lanthanides and radioactive actinides; the archetypal transition metals, and the physically and chemically weak post-transition metals. Specialized subcategories such as the refractory metals and the noble metals also exist.
Metalloids
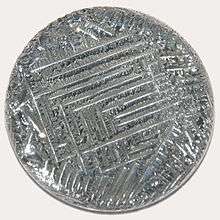
Metalloids are metallic looking brittle solids; tend to share electrons when they react with other substances; have weakly acidic or amphoteric oxides; and are usually found naturally in combined states.
Most are semiconductors, and moderate thermal conductors, and have structures that are more open than those of most metals.
Some metalloids (As, Sb) conduct electricity like metals.
The metalloids, as the smallest major category of elements, are not subdivided further.
Nonmetals
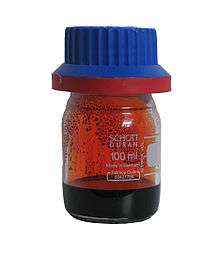
Nonmetals have open structures (unless solidified from gaseous or liquid forms); tend to gain or share electrons when they react with other substances; and do not form distinctly basic oxides.
Most are gases at room temperature; have relatively low densities; are poor electrical and thermal conductors; have relatively high ionisation energies and electronegativities; form acidic oxides; and are found naturally in uncombined states in large amounts.
Some nonmetals (C, black P, S and Se) are brittle solids at room temperature (although each of these also have malleable, pliable or ductile allotropes).
From left to right in the periodic table, the nonmetals can be subdivided into the polyatomic nonmetals which, being nearest to the metalloids, show some incipient metallic character; the diatomic nonmetals, which are essentially nonmetallic; and the monatomic noble gases, which are almost completely inert.
Comparison of properties
Resemble metals | Relatively distinctive | Resemble nonmetals | |||||||||||||||||||||||||||||||||||||||||||||||||||||||||||||||||||||||||||||||||||||||||||||||||||
---|---|---|---|---|---|---|---|---|---|---|---|---|---|---|---|---|---|---|---|---|---|---|---|---|---|---|---|---|---|---|---|---|---|---|---|---|---|---|---|---|---|---|---|---|---|---|---|---|---|---|---|---|---|---|---|---|---|---|---|---|---|---|---|---|---|---|---|---|---|---|---|---|---|---|---|---|---|---|---|---|---|---|---|---|---|---|---|---|---|---|---|---|---|---|---|---|---|---|---|---|---|
Properties compared: | (36) | 6 (17%) | 25 | (69%) | 5 (14%) | ||||||||||||||||||||||||||||||||||||||||||||||||||||||||||||||||||||||||||||||||||||||||||||||||
Physical | (20) | 4 (20%) | 14 | (70%) | 2 (10%) | ||||||||||||||||||||||||||||||||||||||||||||||||||||||||||||||||||||||||||||||||||||||||||||||||
• Presentation & structure | (10) | 2 | 6 | 2 (20%) | |||||||||||||||||||||||||||||||||||||||||||||||||||||||||||||||||||||||||||||||||||||||||||||||||
• Electron-related | (6) | 1 | 5 | ||||||||||||||||||||||||||||||||||||||||||||||||||||||||||||||||||||||||||||||||||||||||||||||||||
• Thermodynamics | (4) | 1 | 3 | ||||||||||||||||||||||||||||||||||||||||||||||||||||||||||||||||||||||||||||||||||||||||||||||||||
Chemical | (16) | 2 (13%) | 11 | (69%) | 3 (19%) | ||||||||||||||||||||||||||||||||||||||||||||||||||||||||||||||||||||||||||||||||||||||||||||||||
• Elemental chemistry | (6) | 3 | 3 (50%) | ||||||||||||||||||||||||||||||||||||||||||||||||||||||||||||||||||||||||||||||||||||||||||||||||||
• Combined form chemistry | (6) | 2 | 4 | ||||||||||||||||||||||||||||||||||||||||||||||||||||||||||||||||||||||||||||||||||||||||||||||||||
• Environmental chemistry | (4) | 4 | |||||||||||||||||||||||||||||||||||||||||||||||||||||||||||||||||||||||||||||||||||||||||||||||||||
The characteristic properties of metals and nonmetals are quite distinct, as shown in the table below. Metalloids, straddling the metal-nonmetal border, are mostly distinct from either, but in a few properties resemble one or the other, as shown in the shading of the metalloid column below and summarized in the small table at the top of this section.
Authors differ in where they divide metals from nonmetals and in whether they recognize an intermediate metalloid category. Some authors count metalloids as nonmetals with weakly nonmetallic properties.[n 1] Others count some of the metalloids as post-transition metals with weakly metallic properties.
Metals[7] | Metalloids | Nonmetals[7] | |
---|---|---|---|
Presentation and structure | (top of section) | ||
Colour | • nearly all are shiny and grey-white • Cu, Cs, Au shiny and golden[8] |
• shiny and grey-white[9] | • most are colourless or dull red, yellow, green, or intermediate shades[10] • C, P, Se, I shiny and grey-white |
Reflectivity | • intermediate to typically high[11][12] | • intermediate[13][14] | • zero or low (mostly)[15] to intermediate[16] |
Form | • almost all solid • Rb, Cs, Fr, Ga, Hg liquid at/near stp[17][18][n 3] |
• all solid[9] | • most are gases[20] • C, P, S, Se, I solid; Br liquid |
Density | • generally high, with some exceptions such as the alkali metals[21] | • lower than neighbouring metals but higher than neighbouring nonmetals[22] | • often low |
Deformability (as a solid) | • most are ductile and malleable • some are brittle (Cr,Mn,Ga,Ru,W,Os,Bi)[23][n 4] |
• brittle[26] | • brittle, when solid • some (C, P, S, Se) have non-brittle forms[n 5] |
Poisson's ratio[n 6] | • low to high[n 7] | • low to intermediate[n 8] | • low to intermediate[n 9] |
Crystalline structure at freezing point[46] | • most are hexagonal or cubic • Ga, U, Np orthorhombic; In, Sn, Pa tetragonal; Sm, Hg, Bi rhombohedral; Pu monoclinic |
• B, As, Sb rhombohedral • Si, Ge cubic • Te hexagonal |
• H, He, C, N, Se hexagonal • O, F, Ne, P, Ar, Kr, Xe, Rn cubic • S, Cl, Br, I orthorhombic |
Packing & coordination number | • close-packed crystal structures[47] • high coordination numbers |
• relatively open crystal structures[48] • medium coordination numbers[49] |
• open structures[50] • low coordination numbers |
Atomic radius (calculated)[51] |
• intermediate to very large • 112–298 pm, average 187 |
• small to intermediate: B, Si, Ge, As, Sb, Te • 87–123 pm, av. 115.5 |
• very small to intermediate • 31–120 pm, av. 76.4 |
Allotropes[52][n 10] | • around half form allotropes • one (Sn) has a metalloid-like allotrope (grey Sn, which forms below 13.2 °C[53]) |
• all or nearly all form allotropes • some (e.g. red B, yellow As) are more nonmetallic in nature |
• some form allotropes • some (e.g. graphitic C, black P, grey Se) are more metalloidal or metallic in nature |
Electron-related | (top of section) | ||
Periodic table block | • s, p, d, f [54] | • p [55] | • s, p [55] |
Outer s and p electrons | • few in number (1–3) • except 0(Pd); 4(Sn,Pb,Fl); 5(Bi); 6(Po) |
• medium number (3–6) | • high number (4–8) • except 1(H); 2(He) |
Electron bands: (valence, conduction) | • nearly all have substantial band overlap • Bi has slight band overlap (semimetal) |
• most have narrow band gap (semiconductors) • As, Sb are semimetals |
• most have wide band gap (insulators) • C (graphite) is a semimetal • P (black), Se, I are semiconductors |
Electron behaviour | • "free" electrons (facilitating electrical and thermal conductivity) | • valence electrons less freely delocalized; considerable covalent bonding present[56] • have Goldhammer-Herzfeld criterion[n 11] ratios straddling unity[60][61] |
• no, few, or directionally confined "free" electrons (generally hampering electrical and thermal conductivity) |
Electrical conductivity | • good to high[n 12] | • intermediate[63] to good[n 13] | • poor to good[n 14] |
... as a liquid[69] | • falls gradually as temperature rises[n 15] | • most behave like metals[60][71] | • increases as temperature rises |
Thermodynamics | (top of section) | ||
Thermal conductivity | • medium to high[72] | • mostly intermediate;[26][73] Si is high | • almost negligible[74] to very high[75] |
Temperature coefficient of resistance[n 16] | • nearly all positive (Pu is negative)[76] | • negative (B, Si, Ge, Te)[77] or positive (As, Sb)[78] | • nearly all negative (C, as graphite, is positive in the direction of its planes)[79][80] |
Melting behaviour | • volume generally expands[81] | • some contract, unlike (most)[82] metals[83] | • volume generally expands[81] |
Enthalpy of fusion | • low to high | • intermediate to very high | • very low to low (except C is very high) |
Elemental chemistry | (top of section) | ||
Overall behaviour | • metallic | • nonmetallic[84] | • nonmetallic |
Ion formation | • tend to form cations | • some tendency to form anions in water[6] • solution chemistry dominated by formation and reactions of oxyanions[85][86] |
• tend to form anions |
Bonds | • seldom form covalent compounds | • form salts as well as covalent compounds[87] | • form many covalent compounds |
Oxidation number | • nearly always positive | • positive or negative[88] | • positive or negative |
Ionization energy | • relatively low | • intermediate[89][90] | • high |
Electronegativity | • usually low | • close to 2,[91] i.e., 1.9–2.2[92][n 17] | • high |
Combined form chemistry | (top of section) | ||
With metals | • form alloys | • can form alloys[87][95][96] | • form ionic or interstitial compounds |
With carbon | • carbides and organometallic compounds | • same as metals | • carbon-nonmetal (e.g. CO2, CS2)[n 18] or organic (e.g. CH4, C6H12O6) compounds |
Hydrides | • ionic, with alkali, alkaline earth metals • metallic, with transition metals • covalent, with post-transition metals |
• covalent, volatile hydrides[97] | • covalent, gaseous or liquid hydrides |
Oxides | • nearly all solid (Mn2O7 is a liquid) • very few glass formers[98] • lower oxides: ionic and basic • higher oxides: more covalent, acidic |
• solid • glass formers (B, Si, Ge, As, Sb, Te)[99] • polymeric in structure;[100] tend to be amphoteric or weakly acidic[9][101] |
• solid, liquid or gaseous • few glass formers (P, S, Se)[102] • covalent, acidic |
Sulfates | • do form[n 19][n 20] | • most form[n 21] | • some form[n 22] |
Halides, esp. chlorides (see also[123]) | • typically ionic, involatile • generally insoluble in organic solvents • mostly water-soluble (not hydrolysed) • more covalent, volatile, and susceptible to hydrolysis[n 23] and organic solvents with higher halogens and weaker metals[124][125] |
• covalent, volatile[126] • usually dissolve in organic solvents[127] • partly or completely hydrolysed[128] • some reversibly hydrolysed[128] |
• covalent, volatile • usually dissolve in organic solvents • generally completely or extensively hydrolyzed • not always susceptible to hydrolysis if parent nonmetal at maximum covalency for period e.g. CF4, SF6 (then nil reaction)[129] |
Environmental chemistry | (top of section) | ||
Molar composition of Earth's ecosphere[n 24] | • about 14%, mostly Al, Na, Ng, Ca, Fe, K | • about 17%, mostly Si | • about 69%, mostly O, H |
Primary form on Earth | • most occur in combined states, as carbonates, silicates, phosphates, oxides, sulfides, or halides • some (e.g. Au, Cu, Ag, Pt) occur in free or uncombined states[133] |
• all occur in combined states, as borates, silicates, sulfides, or tellurides | • elemental C, N, O, S, noble gases are plentiful • H,[n 25] F[n 26], Se occur primarily in compounds • P, Cl, Br, I occur only in compounds, as phosphates, oxides, selenides or halides |
Required by mammals | • large amounts needed: Na, Mg, K, Ca • trace amounts needed of some others |
• trace amounts needed: B, Si, As | • large amounts needed: H, C, N, O, P, S, Cl • trace amounts needed: Se, Br, I, possibly F • only noble gases not needed |
Composition of the human body, by weight | • about 1.5% Ca • traces of most others through 92U |
• trace amounts of B, Si, Ge, As, Sb, Te | • about 97% O, C, H, N, P • others detectable except noble gases |
Anomalous properties
There were exceptions…in the periodic table, anomalies too—some of them profound. Why, for example, was manganese such a bad conductor of electricity, when the elements on either side of it were reasonably good conductors? Why was strong magnetism confined to the iron metals? And yet these exceptions, I was somehow convinced, reflected special additional mechanisms at work…
Oliver Sacks
Uncle Tungsten (2001, p. 204)
Within each category, elements can be found with one or two properties very different from the expected norm, or that are otherwise notable.
Metals
|
Sodium, potassium, rubidium, caesium, barium, platinum, gold
- The common notions that "alkali metal ions (group 1A) always have a +1 charge"[135] and that "transition elements do not form anions"[136] are textbook errors. The synthesis of a crystalline salt of the sodium anion Na− was reported in 1974. Since then further compounds ("alkalides") containing anions of all other alkali metals except Li and Fr, as well as that of Ba, have been prepared. In 1943, Sommer reported the preparation of the yellow transparent compound CsAu. This was subsequently shown to consist of caesium cations (Cs+) and auride anions (Au−) although it was some years before this conclusion was accepted. Several other aurides (KAu, RbAu) have since been synthesized, as well as the red transparent compound Cs2Pt which was found to contain Cs+ and Pt2− ions.[137]
|
- Well-behaved metals have crystal structures featuring unit cells with up to four atoms. Manganese has a complex crystal structure with a 58-atom unit cell, effectively four different atomic radii, and four different coordination numbers (10, 11, 12 and 16). It has been described as resembling "a quaternary intermetallic compound with four Mn atom types bonding as if they were different elements."[138] The half-filled 3d shell of manganese appears to be the cause of the complexity. This confers a large magnetic moment on each atom. Below 727 °C, a unit cell of 58 spatially diverse atoms represents the energetically lowest way of achieving a zero net magnetic moment.[139] The crystal structure of manganese makes it a hard and brittle metal, with low electrical and thermal conductivity. At higher temperatures "greater lattice vibrations nullify magnetic effects"[138] and manganese adopts less complex structures.[140]
|
Iron, cobalt, nickel, gadolinium, terbium, dysprosium
- The only elements strongly attracted to magnets are iron, cobalt, and nickel at room temperature, gadolinium just below, and terbium and dysprosium at ultra cold temperatures (below −54 °C and below −185 °C respectively).[141]
|
|
- The malleability of gold is extraordinary: a fist sized lump can be hammered and separated into one million paper back sized sheets, each 10 nm thick, 1600 times thinner than regular kitchen aluminium foil (0.016 mm thick).
|
- Bricks and bowling balls will float on the surface of mercury thanks to it having a density 13.5 times that of water. Equally, a solid mercury bowling ball would weigh around 50 pounds and, if it could be kept cold enough, would float on the surface of liquid gold.
- The only metal having an ionisation energy higher than some nonmetals (sulfur and selenium) is mercury.
- Mercury and its compounds have a reputation for toxicity but on a scale of 1 to 10, dimethylmercury ((CH3)2Hg) (abbr. DMM), a volatile colourless liquid, has been described as a 15. It is so dangerous that scientists have been encouraged to use less toxic mercury compounds wherever possible. In 1997, Karen Wetterhahn, a professor of chemistry specialising in toxic metal exposure, died of mercury poisoning ten months after a few drops of DMM landed on her "protective" latex gloves. Although Wetterhahn had been following the then published procedures for handling this compound, it passed through her gloves and skin within seconds. It is now known that DMM is exceptionally permeable to (ordinary) gloves, skin and tissues. And its toxicity is such that less than one-tenth of a ml applied to the skin will be seriously toxic.[143]
|
- The expression, to "go down like a lead balloon" is anchored in the common view of lead as a dense, heavy metal—being nearly as dense as mercury. However, it is possible to construct a balloon made of lead foil, filled with a helium and air mixture, which will float and be buoyant enough to carry a small load.
|
- Bismuth has the longest half life of any naturally occurring element; its only primordial isotope, bismuth-209, was found in 2003 to be slightly radioactive, decaying via alpha decay with a half life more than a billion times the estimated age of the universe. Prior to this discovery, Bi-209 was thought to be the heaviest naturally occurring stable isotope;[144] this distinction now belongs to lead.
|
|
- It is a commonly held belief that metals reduce their electrical conductivity when heated. Plutonium increases its electrical conductivity when heated in the temperature range of around –175 to +125 °C.
Metalloids
|
- Boron is the only element with a partially disordered structure in its most thermodynamically stable crystalline form.[148]
|
- These elements are record holders within the field of superacid chemistry. For seven decades, fluorosulfonic acid HSO3F and trifluoromethanesulfonic acid CF3SO3H were the strongest known acids that could be isolated as single compounds. Both are about a thousand times more acidic than pure sulfuric acid. In 2004, a boron compound broke this record by a thousand fold with the synthesis of carborane acid H(CHB11Cl11). Another metalloid, antimony, features in the strongest known acid, a mixture 10 billion times stronger than carborane acid. This is fluoroantimonic acid H2F[SbF6], a mixture of antimony pentafluoride SbF5 and hydrofluoric acid HF.
|
- The thermal conductivity of silicon is better than that of most metals.
- A sponge-like porous form of silicon (p-Si) is typically prepared by the electrochemical etching of silicon wafers in a hydrofluoric acid solution.[149] Flakes of p-Si sometimes appear red;[150] it has a band gap of 1.97–2.1 eV.[151] The many tiny pores in porous silicon give it an enormous internal surface area, up to 1,000 m2/cm3.[152] When exposed to an oxidant,[153] especially a liquid oxidant,[152] the high surface-area to volume ratio of p-Si creates a very efficient burn, accompanied by nano-explosions,[149] and sometimes by ball-lightning-like plasmoids with, for example, a diameter of 0.1–0.8 m, a velocity of up to 0.5 m/s and a lifetime of up to 1s.[154] The first ever spectrographic analysis of a ball lightning event (in 2012) revealed the presence of silicon, iron and calcium, these elements also being present in the soil.[155]
|
- Metals are said to be fusible, resulting in some confusion in old chemistry as to whether arsenic was a true metal, or a nonmetal, or something in between. It sublimes rather than melts at standard atmospheric pressure, like the nonmetals carbon and red phosphorus.
|
- A high-energy explosive form of antimony was first obtained in 1858. It is prepared by the electrolysis of any of the heavier antimony trihalides (SbCl3, SbBr3, SbI3) in a hydrochloric acid solution at low temperature. It comprises amorphous antimony with some occluded antimony trihalide (7–20% in the case of the trichloride). When scratched, struck, powdered or heated quickly to 200 °C, it "flares up, emits sparks and is converted explosively into the lower-energy, crystalline grey antimony."[156]
Nonmetals
|
- Water (H2O), a well known oxide of hydrogen, is a spectacular anomaly.[157] Extrapolating from the heavier hydrogen chalcogenides, namely hydrogen sulfide H2S, hydrogen selenide H2Se, and hydrogen telluride H2Te, water should be "a foul-smelling, poisonous, inflammable gas…condensing to a nasty liquid [at] around –100° C". Instead, due to hydrogen bonding, water is "stable, potable, odorless, benign, and…indispensable to life".[158]
- Less well known of the oxides of hydrogen is the trioxide, H2O3. Berthelot proposed the existence of this oxide in 1880 but his suggestion was soon forgotten as there was no way of testing it using the technology of the time.[159] Hydrogen trioxide was prepared in 1994 by replacing the oxygen used in the industrial process for making hydrogen peroxide, with ozone. The yield is about 40 per cent, at –78 °C; above around –40 °C it decomposes into water and oxygen.[160] Derivatives of hydrogen trioxide, such as F3C–O–O–O–CF3 ("bis(trifluoromethyl) trioxide") are known; these are metastable at room temperature.[161] Mendeleev went a step further, in 1895, and proposed the existence of hydrogen tetroxide HO–O–O–OH as a transient intermediate in the decomposition of hydrogen peroxide;[159] this was prepared and characterised in 1974, using a matrix isolation technique. Alkali metal ozonide salts of the unknown hydrogen ozonide (HO3) are also known; these have the formula MO3.[161]
|
- At temperatures below 0.3 and 0.8 K respectively, helium-3 and helium-4 each have a negative enthalpy of fusion. This means that, at the appropriate constant pressures, these substances freeze with the addition of heat.
- Until 1999 helium was thought to be too small to form a cage clathrate—a compound in which a guest atom or molecule is encapsulated in a cage formed by a host molecule—at atmospheric pressure. In that year the synthesis of microgram quantities of He@C20H20 represented the first such helium clathrate and (what was described as) the world's smallest helium balloon.[162]
|
- Graphite is the most electrically conductive nonmetal, better than some metals.
- Diamond is the best natural conductor of heat; it even feels cold to the touch. Its thermal conductivity (2,200 W/m•K) is five times greater than the most conductive metal (Ag at 429); 300 times higher than the least conductive metal (Pu at 6.74); and nearly 4,000 times that of water (0.58) and 100,000 times that of air (0.0224). This high thermal conductivity is used by jewelers and gemologists to separate diamonds from imitations.
- Graphene aerogel, produced in 2012 by freeze-drying a solution of carbon nanotubes and graphite oxide sheets and chemically removing oxygen, is seven times lighter than air, and ten per cent lighter than helium. It is the lightest solid known (0.16 mg/cm3), conductive and elastic.[163]
|
- The least stable and most reactive form of phosphorus is the white allotrope. It is a hazardous, highly flammable and toxic substance, spontaneously igniting in air and producing phosphoric acid residue. It is therefore normally stored under water. White phosphorus is also the most common, industrially important, and easily reproducible allotrope, and for these reasons is regarded as the standard state of phosphorus. The most stable form is the black allotrope, which is a metallic looking, brittle and relatively non-reactive semiconductor (unlike the white allotrope, which has a white or yellowish appearance, is pliable, highly reactive and a semiconductor). When assessing periodicity in the physical properties of the elements it needs to be borne in mind that the quoted properties of phosphorus tend to be those of its least stable form rather than, as is the case with all other elements, the most stable form.
|
- The mildest of the halogens, iodine is the active ingredient in tincture of iodine, a disinfectant. This can be found in household medicine cabinets or emergency survival kits. Tincture of iodine will rapidly dissolve gold,[164] a task ordinarily requiring the use of aqua regia (a highly corrosive mixture of nitric and hydrochloric acids).
Notes
- ↑ For example:
- Brinkley[2] writes that boron has weakly nonmetallic properties.
- Glinka[3] describes silicon as a weak nonmetal.
- Eby et al.[4] discuss the weak chemical behaviour of the elements close to the metal-nonmetal borderline.
- Booth and Bloom[5] say "A period represents a stepwise change from elements strongly metallic to weakly metallic to weakly nonmetallic to strongly nonmetallic, and then, at the end, to an abrupt cessation of almost all chemical properties ...".
- Cox[6] notes "nonmetallic elements close to the metallic borderline (Si, Ge, As, Sb, Se, Te) show less tendency to anionic behaviour and are sometimes called metalloids."
- ↑ At standard pressure and temperature, for the elements in their most thermodynamically stable forms, unless otherwise noted
- ↑ Copernicium is reported to be the only metal known to be a gas at room temperature.[19]
- ↑ Whether polonium is ductile or brittle is unclear. It is predicted to be ductile based on its calculated elastic constants.[24] It has a simple cubic crystalline structure. Such a structure has few slip systems and "leads to very low ductility and hence low fracture resistance".[25]
- ↑ Carbon as exfoliated (expanded) graphite,[27] and as metre-long carbon nanotube wire;[28] phosphorus as white phosphorus (soft as wax, pliable and can be cut with a knife, at room temperature);[29] sulfur as plastic sulfur;[30] and selenium as selenium wires.[31]
- ↑ For polycrystalline forms of the elements unless otherwise noted. Determining Poisson's ratio accurately is a difficult proposition and there could be considerable uncertainty in some reported values.[32]
- ↑ Beryllium has the lowest known value (0.0476) amongst elemental metals; indium and thallium each have the highest known value (0.46). Around one third show a value ≥ 0.33.[33]
- ↑ Boron 0.13;[34] silicon 0.22;[35] germanium 0.278;[36] amorphous arsenic 0.27;[37] antimony 0.25;[38] tellurium ~0.2.[39]
- ↑ Graphitic carbon 0.25;[40] [diamond 0.0718];[41] black phosphorus 0.30;[42] sulfur 0.287;[43] amorphous selenium 0.32;[44] amorphous iodine ~0.[45]
- ↑ At atmospheric pressure, for elements with known structures
- ↑ The Goldhammer-Herzfeld criterion is a ratio that compares the force holding an individual atom's valence electrons in place with the forces, acting on the same electrons, arising from interactions between the atoms in the solid or liquid element. When the interatomic forces are greater than or equal to the atomic force, valence electron itinerancy is indicated. Metallic behaviour is then predicted.[57] Otherwise nonmetallic behaviour is anticipated. The Goldhammer-Herzfeld criterion is based on classical arguments.[58] It nevertheless offers a relatively simple first order rationalization for the occurrence of metallic character amongst the elements.[59]
- ↑ Metals have electrical conductivity values of from 6.9 × 103 S•cm−1 for manganese to 6.3 × 105 for silver.[62]
- ↑ Metalloids have electrical conductivity values of from 1.5 × 10−6 S•cm−1 for boron to 3.9 × 104 for arsenic.[64] If selenium is included as a metalloid the applicable conductivity range would start from ~10−9 to 10−12 S•cm−1.[65][66][67]
- ↑ Nonmetals have electrical conductivity values of from ~10−18 S•cm−1 for the elemental gases to 3 × 104 in graphite.[68]
- ↑ Mott and Davis[70] note however that 'liquid europium has a negative temperature coefficient of resistance' i.e. that conductivity increases with rising temperature
- ↑ At or near room temperature
- ↑ Chedd[93] defines metalloids as having electronegativity values of 1.8 to 2.2 (Allred-Rochow scale). He included boron, silicon, germanium, arsenic, antimony, tellurium, polonium and astatine in this category. In reviewing Chedd's work, Adler[94] described this choice as arbitrary, given other elements have electronegativities in this range, including copper, silver, phosphorus, mercury and bismuth. He went on to suggest defining a metalloid simply as, 'a semiconductor or semimetal' and 'to have included the interesting materials bismuth and selenium in the book'.
- ↑ Phosphorus is known to form a carbide in thin films.
- ↑ See, for example, the sulfates of the transition metals,[103] the lanthanides[104] and the actinides.[105]
- ↑ Sulfates of osmium have not been characterized with any great degree of certainty.[106]
- ↑ Common metalloids: Boron is reported to be capable of forming an oxysulfate (BO)2SO4,[107] a bisulfate B(HSO4)3[108] and a sulfate B2(SO4)3.[109] The existence of a sulfate has been disputed.[110] In light of the existence of silicon phosphate, a silicon sulfate might also exist.[111] Germanium forms an unstable sulfate Ge(SO4)2 (d 200 °C).[112] Arsenic forms oxide sulfates As2O(SO4)2 (= As2O3.2SO3)[113] and As2(SO4)3 (= As2O3.3SO3).[114] Antimony forms a sulfate Sb2(SO4)3 and an oxysulfate (SbO)2SO4.[115] Tellurium forms an oxide sulfate Te2O3(SO)4.[116] Less common: Polonium forms a sulfate Po(SO4)2.[117] It has been suggested that the astatine cation forms a weak complex with sulfate ions in acidic solutions.[118]
- ↑ Hydrogen forms hydrogen sulfate H2SO4. Carbon forms (a blue) graphite hydrogen sulfate C+
24HSO–
4 • 2.4H2SO4.[119] Nitrogen forms nitrosyl hydrogen sulfate (NO)HSO4 and nitronium (or nitryl) hydrogen sulfate (NO2)HSO4.[120] There are indications of a basic sulfate of selenium SeO2.SO3 or SeO(SO4).[121] Iodine forms a polymeric yellow sulfate (IO)2SO4.[122] - ↑ layer-lattice types often reversibly so
- ↑ Based on a table of the elemental composition of the biosphere, and lithosphere (crust, atmosphere, and seawater) in Georgievskii,[130] and the masses of the crust and hydrosphere give in Lide and Frederikse.[131] The mass of the biosphere is negligible, having a mass of about one billionth that of the lithosphere. "The oceans constitute about 98 percent of the hydrosphere, and thus the average composition of the hydrosphere is, for all practical purposes, that of seawater."[132]
- ↑ Hydrogen gas is produced by some bacteria and algae and is a natural component of flatus. It can be found in the Earth's atmosphere at a concentration of 1 part per million by volume.
- ↑ Fluorine can be found in its elemental form, as an occlusion in the mineral antozonite[134]
- ↑ In 1934, a team led by Enrico Fermi postulated that transuranic elements may have been produced as a result of bombarding uranium with neutrons, a finding which was widely accepted for a few years. In the same year Ida Noddack, a German scientist and subsequently a three-time Nobel prize nominee, criticised this assumption, writing "It is conceivable that the nucleus breaks up into several large fragments, which would of course be isotopes of known elements but would not be neighbors of the irradiated element."[146][emphasis added] In this, Noddak defied the understanding of the time without offering experimental proof or theoretical basis, but nevertheless presaged what would be known a few years later as nuclear fission. Her paper was generally ignored as, in 1925, she and two colleagues claimed to have discovered element 43, then proposed to be called masurium (later discovered in 1936 by Perrier and Segrè, and named technetium). Had Ida Noddack's paper been accepted it is likely that Germany would have had an atomic bomb and, 'the history of the world would have been [very] different.'[147]
Citations
- ↑ Mendeléeff 1897, p. 274
- ↑ Brinkley 1945, p. 378
- ↑ Glinka 1965, p. 88
- ↑ Eby et al. 1943, p. 404
- ↑ Booth & Bloom 1972, p. 426
- 1 2 Cox 2004, p. 27
- 1 2 Kneen, Rogers & Simpson, 1972, p. 263. Columns 2 and 4 are sourced from this reference unless otherwise indicated.
- ↑ Russell & Lee 2005, p. 147
- 1 2 3 Rochow 1966, p. 4
- ↑ Pottenger & Bowes 1976, p. 138
- ↑ Askeland, Fulay & Wright 2011, p. 806
- ↑ Born & Wolf 1999, p. 746
- ↑ Lagrenaudie 1953
- ↑ Rochow 1966, pp. 23, 25
- ↑ Burakowski & Wierzchoń 1999, p. 336
- ↑ Olechna & Knox 1965, pp. A991‒92
- ↑ Stoker 2010, p. 62
- ↑ Chang 2002, p. 304. Chang speculates that the melting point of francium would be about 23 °C.
- ↑ New Scientist 1975; Soverna 2004; Eichler, Aksenov & Belozeroz et al. 2007; Austen 2012
- ↑ Hunt 2000, p. 256
- ↑ Sisler 1973, p. 89
- ↑ Hérold 2006, pp. 149–150
- ↑ Russell & Lee 2005
- ↑ Legit, Friák & Šob 2010, p. 214118-18
- ↑ Manson & Halford 2006, pp. 378, 410
- 1 2 McQuarrie & Rock 1987, p. 85
- ↑ Chung 1987; Godfrin & Lauter 1995
- ↑ Cambridge Enterprise 2013
- ↑ Faraday 1853, p. 42; Holderness & Berry 1979, p. 255
- ↑ Partington 1944, p. 405
- ↑ Regnault 1853, p. 208
- ↑ Christensen 2012, p. 14
- ↑ Gschneidner 1964, pp. 292‒93.
- ↑ Qin et al. 2012, p. 258
- ↑ Hopcroft, Nix & Kenny 2010, p. 236
- ↑ Greaves et al. 2011, p. 826
- ↑ Brassington et al. 1980
- ↑ Martienssen & Warlimont 2005, p. 100
- ↑ Witczak 2000, p. 823
- ↑ Marlowe 1970, p. 6;Slyh 1955, p. 146
- ↑ Klein & Cardinale 1992, pp. 184‒85
- ↑ Appalakondaiah et al. 2012, pp. 035105‒6
- ↑ Sundara Rao 1950; Sundara Rao 1954; Ravindran 1998, pp. 4897‒98
- ↑ Lindegaard & Dahle 1966, p. 264
- ↑ Leith 1966, pp. 38‒39
- ↑ Donohoe 1982; Russell & Lee 2005
- ↑ Gupta et al. 2005, p. 502
- ↑ Walker, Newman & Enache 2013, p. 25
- ↑ Wiberg 2001, p. 143
- ↑ Batsanov & Batsanov 2012, p. 275
- ↑ Clementi & Raimondi 1963; Clementi, Raimondi & Reinhardt 1967
- ↑ Addison 1964; Donohoe 1982
- ↑ Vernon 2013, p. 1704
- ↑ Parish 1977, pp. 34, 48, 112, 142, 156, 178
- 1 2 Emsley 2001, p. 12
- ↑ Russell 1981, p. 628
- ↑ Herzfeld 1927; Edwards 2000, pp. 100–103
- ↑ Edwards 1999, p. 416
- ↑ Edwards & Sienko 1983, p. 695
- 1 2 Edwards & Sienko 1983, p. 691
- ↑ Edwards et al. 2010
- ↑ Desai, James & Ho 1984, p. 1160; Matula 1979, p. 1260
- ↑ Choppin & Johnsen 1972, p. 351
- ↑ Schaefer 1968, p. 76; Carapella 1968, p. 30
- ↑ Glazov, Chizhevskaya & Glagoleva 1969 p. 86
- ↑ Kozyrev 1959, p. 104
- ↑ Chizhikov & Shchastlivyi 1968, p. 25
- ↑ Bogoroditskii & Pasynkov 1967, p. 77; Jenkins & Kawamura 1976, p. 88
- ↑ Rao & Ganguly 1986
- ↑ Mott & Davis 2012, p. 177
- ↑ Anita 1998
- ↑ Cverna 2002, p.1
- ↑ Cordes & Scaheffer 1973, p. 79
- ↑ Hill & Holman 2000, p. 42
- ↑ Tilley 2004, p. 487
- ↑ Russell & Lee 2005, p. 466
- ↑ Orton 2004, pp. 11–12
- ↑ Zhigal'skii & Jones 2003, p. 66: 'Bismuth, antimony, arsenic and graphite are considered to be semimetals ... In bulk semimetals ... the resistivity will increase with temperature ... to give a positive temperature coefficient of resistivity ...'
- ↑ Jauncey 1948, p. 500: 'Nonmetals mostly have negative temperature coefficients. For instance, carbon ... [has a] resistance [that] decreases with a rise in temperature. However, recent experiments on very pure graphite, which is a form of carbon, have shown that pure carbon in this form behaves similarly to metals in regard to its resistance.'
- ↑ Reynolds 1969, pp. 91–92
- 1 2 Wilson 1966, p. 260
- ↑ Wittenberg 1972, p. 4526
- ↑ Habashi 2003, p. 73
- ↑ Bailar et al. 1989, p. 742
- ↑ Hiller & Herber 1960, inside front cover; p. 225
- ↑ Beveridge et al. 1997, p. 185
- 1 2 Young & Sessine 2000, p. 849
- ↑ Bailar et al. 1989, p. 417
- ↑ Metcalfe, Williams & Castka 1966, p. 72
- ↑ Chang 1994, p. 311
- ↑ Pauling 1988, p. 183
- ↑ Mann et al. 2000, p. 2783
- ↑ Chedd 1969, pp. 24–25
- ↑ Adler 1969, pp. 18–19
- ↑ Hultgren 1966, p. 648
- ↑ Bassett et al. 1966, p. 602
- ↑ Rochow 1966, p. 34
- ↑ Martienssen & Warlimont 2005, p. 257
- ↑ Sidorov 1960
- ↑ Brasted 1974, p. 814
- ↑ Atkins 2006 et al., pp. 8, 122–23
- ↑ Rao 2002, p. 22
- ↑ Wickleder, Pley & Büchner 2006; Betke & Wickleder 2011
- ↑ Cotton 1994, p. 3606
- ↑ Keogh 2005, p. 16
- ↑ Raub & Griffith 1980, p. 167
- ↑ Nemodruk & Karalova 1969, p. 48
- ↑ Sneed 1954, p. 472; Gillespie & Robinson 1959, p. 407
- ↑ Zuckerman & Hagen 1991, p. 303
- ↑ Sanderson 1967, p. 178
- ↑ Iler 1979, p. 190
- ↑ Sanderson 1960, p. 162; Greenwood & Earnshaw 2002, p. 387
- ↑ Mercier & Douglade 1982
- ↑ Douglade & Mercier 1982
- ↑ Wiberg 2001, p. 764
- ↑ Wickleder 2007, p. 350
- ↑ Bagnall 1966, pp. 140−41
- ↑ Berei & Vasáros 1985, pp. 221, 229
- ↑ Wiberg 2001, p. 795
- ↑ Lidin 1996, pp. 266, 270; Brescia et al. 1975, p. 453
- ↑ Greenwood & Earnshaw 2002, p. 786
- ↑ Furuseth et al. 1974
- ↑ Holtzclaw, Robinson & Odom 1991, pp. 706–07; Keenan, Kleinfelter & Wood 1980, pp. 693–95
- ↑ Kneen, Rogers & Simpson 1972, p. 278
- ↑ Heslop & Robinson 1963, p. 417
- ↑ Rochow 1966, pp. 28–29
- ↑ Bagnall 1966, pp. 108, 120; Lidin 1996, passim
- 1 2 Smith 1921, p. 295; Sidgwick 1950, pp. 605, 608; Dunstan 1968, pp. 408, 438
- ↑ Dunstan 1968, pp. 312, 408
- ↑ Georgievskii 1982, p. 58
- ↑ Lide & Frederikse 1998, p. 14–6
- ↑ Hem 1985, p. 7
- ↑ Perkins 1998, p. 350
- ↑ Sanderson 2012
- ↑ Brown et al. 2009, p. 137
- ↑ Bresica et al. 1975, p. 137
- ↑ Jansen, Martin (2005-11-30). "Effects of relativistic motion of electrons on the chemistry of gold and platinum". Solid State Sciences. 7 (12): 1464–1474. doi:10.1016/j.solidstatesciences.2005.06.015.
- 1 2 Russell & Lee 2005, p. 246
- ↑ Russell & Lee 2005, p. 244–5
- ↑ Donohoe 1982, pp. 191–196; Russell & Lee 2005, pp. 244–247
- ↑ Jackson, Mike (2000). "Wherefore Gadolinium? Magnetism of the Rare Earths" (PDF). IRM Quarterly. Institute for Rock Magnetism. 10 (3): 6.
- ↑ Stoye 2014
- ↑ Witt 1991; Endicott 1998
- ↑ Dumé, Belle (23 April 2003). "Bismuth breaks half-life record for alpha decay". Physicsworld.
- ↑ Benedict et al. 1946, p. 19
- ↑ Noddack 1934, p. 653
- ↑ Sacks 2001, p. 205: 'This story was told by Glenn Seaborg when he was presenting his recollections at a conference in November 1997.'
- ↑ Dalhouse University 2015; White et al. 2015
- 1 2 DuPlessis 2007, p. 133
- ↑ Gösele & Lehmann 1994, p. 19
- ↑ Chen, Lee & Bosman 1994
- 1 2 Kovalev et al. 2001, p. 068301-1
- ↑ Mikulec, Kirtland & Sailor 2002
- ↑ Bychkov 2012, pp. 20–21; see also Lazaruk et al. 2007
- ↑ Slezak 2014
- ↑ Wiberg 2001, p. 758; see also Fraden 1951
- ↑ Sacks 2001, p. 204
- ↑ Sacks 2001, pp. 204–205
- 1 2 Cerkovnik & Plesničar 2013, p. 7930
- ↑ Emsley 1994, p. 1910
- 1 2 Wiberg 2001, p. 497
- ↑ Cross, Saunders & Prinzbach; Chemistry Views 2015
- ↑ Sun, Xu & Gao 2013; Anthony 2013
- ↑ Nakao 1992
References
- Addison WE 1964, The allotropy of the elements, Oldbourne Press, London
- Adler D 1969, 'Half-way elements: The technology of metalloids', book review, Technology Review, vol. 72, no. 1, Oct/Nov, pp. 18–19
- Anita M 1998, 'Focus: Levitating Liquid Boron', American Physical Society, viewed 14 December 2014
- Anthony S 2013, 'Graphene aerogel is seven times lighter than air, can balance on a blade of grass', ExtremeTech, April 10, accessed 8 February 2015
- Appalakondaiah S, Vaitheeswaran G, Lebègue S, Christensen NE & Svane A 2012, 'Effect of van der Waals interactions on the structural and elastic properties of black phosphorus,' Physical Review B, vol. 86, pp. 035105‒1 to 9, doi:10.1103/PhysRevB.86.035105
- Askeland DR, Fulay PP & Wright JW 2011, The science and engineering of materials, 6th ed., Cengage Learning, Stamford, CT, ISBN 0-495-66802-8
- Atkins P, Overton T, Rourke J, Weller M & Armstrong F 2006, Shriver & Atkins' inorganic chemistry, 4th ed., Oxford University Press, Oxford, ISBN 0-7167-4878-9
- Austen K 2012, 'A factory for elements that barely exist', NewScientist, 21 Apr, p. 12, ISSN 1032-1233
- Bagnall KW 1966, The chemistry of selenium, tellurium and polonium, Elsevier, Amsterdam
- Bailar JC, Moeller T, Kleinberg J, Guss CO, Castellion ME & Metz C 1989, Chemistry, 3rd ed., Harcourt Brace Jovanovich, San Diego, ISBN 0-15-506456-8
- Bassett LG, Bunce SC, Carter AE, Clark HM & Hollinger HB 1966, Principles of chemistry, Prentice-Hall, Englewood Cliffs, NJ
- Batsanov SS & Batsanov AS 2012, Introduction to structural chemistry, Springer Science+Business Media, Dordrecht, ISBN 978-94-007-4770-8
- Benedict M, Alvarez LW, Bliss LA, English SG, Kinzell AB, Morrison P, English FH, Starr C & Williams WJ 1946, 'Technological control of atomic energy activities', "Bulletin of the Atomic Scientists," vol. 2, no. 11, pp. 18–29
- Berei K & Vasáros L 1985, 'Astatine compounds', in Kugler & Keller
- Betke U & Wickleder MS 2011, 'Sulfates of the refractory metals: Crystal structure and thermal behavior of Nb2O2(SO4)3, MoO2(SO4), WO(SO4)2, and two modifications of Re2O5(SO4)2', Inorganic chemistry, vol. 50, no. 3, pp 858–872, doi:10.1021/ic101455z
- Beveridge TJ, Hughes MN, Lee H, Leung KT, Poole RK, Savvaidis I, Silver S & Trevors JT 1997, 'Metal–microbe interactions: Contemporary approaches', in RK Poole (ed.), Advances in microbial physiology, vol. 38, Academic Press, San Diego, pp. 177–243, ISBN 0-12-027738-7
- Bogoroditskii NP & Pasynkov VV 1967, Radio and electronic materials, Iliffe Books, London
- Booth VH & Bloom ML 1972, Physical science: a study of matter and energy, Macmillan, New York
- Born M & Wolf E 1999, Principles of optics: Electromagnetic theory of propagation, interference and diffraction of light, 7th ed., Cambridge University Press, Cambridge, ISBN 0-521-64222-1
- Brassington MP, Lambson WA, Miller AJ, Saunders GA & Yogurtçu YK 1980, 'The second- and third-order elastic constants of amorphous arsenic', Philosophical Magazine Part B, vol. 42, no. 1., pp. 127–148, doi:10.1080/01418638008225644
- Brasted RC 1974, 'Oxygen group elements and their compounds', in The new Encyclopædia Britannica, vol. 13, Encyclopædia Britannica, Chicago, pp. 809–824
- Brescia F, Arents J, Meislich H & Turk A 1975, Fundamentals of chemistry, 3rd ed., Academic Press, New York, p. 453, ISBN 978-0-12-132372-1
- Brinkley SR 1945, Introductory general chemistry, 3rd ed., Macmillan, New York
- Brown TL, LeMay HE, Bursten BE, Murphy CJ & Woodward P 2009, Chemistry: The Central Science, 11th ed., Pearson Education, New Jersey, ISBN 978-0-13-235-848-4
- Burakowski T & Wierzchoń T 1999, Surface engineering of metals: Principles, equipment, technologies, CRC Press, Boca Raton, Fla, ISBN 0-8493-8225-4
- Bychkov VL 2012, 'Unsolved Mystery of Ball Lightning', in Atomic Processes in Basic and Applied Physics, V Shevelko & H Tawara (eds), Springer Science & Business Media, Heidelberg, pp. 3–24, ISBN 978-3-642-25568-7
- Carapella SC 1968a, 'Arsenic' in CA Hampel (ed.), The encyclopedia of the chemical elements, Reinhold, New York, pp. 29–32
- Cerkovnik J & Plesničar B 2013, 'Recent Advances in the Chemistry of Hydrogen Trioxide (HOOOH), Chemical Reviews, vol. 113, no. 10), pp. 7930–7951, doi:10.1021/cr300512s
- Chang R 1994, Chemistry, 5th (international) ed., McGraw-Hill, New York
- Chang R 2002, Chemistry, 7th ed., McGraw Hill, Boston
- Chedd G 1969, Half-way elements: The technology of metalloids, Doubleday, New York
- Chen Z, Lee T-Y & Bosman G 1994, 'Electrical Band Gap of Porous Silicon', Applied Physics Letters, vol. 64, p. 3446, doi:10.1063/1.111237
- Chizhikov DM & Shchastlivyi VP 1968, Selenium and selenides, translated from the Russian by EM Elkin, Collet's, London
- Choppin GR & Johnsen RH 1972, Introductory chemistry, Addison-Wesley, Reading, Massachusetts
- Christensen RM 2012, 'Are the elements ductile or brittle: A nanoscale evaluation,' in Failure theory for materials science and engineering, chapter 12, p. 14
- Clementi E & Raimondi DL 1963, Atomic Screening Constants from SCF Functions, Journal of Chemical Physics, vol. 38, pp. 2868–2689, doi:10.1063/1.1733573
- Clementi E, Raimondi DL & Reinhardt WP 1967, 'Atomic Screening Constants from SCF Functions. II. Atoms with 37 to 86 Electrons', Journal of Chemical Physics, vol. 47, pp. 1300–1306, doi:10.1063/1.1712084
- Cordes EH & Scaheffer R 1973, Chemistry, Harper & Row, New York
- Cotton SA 1994, 'Scandium, yttrium & the lanthanides: Inorganic & coordination chemistry', in RB King (ed.), Encyclopedia of inorganic chemistry, 2nd ed., vol. 7, John Wiley & Sons, New York, pp. 3595–3616, ISBN 978-0-470-86078-6
- Cox PA 2004, Inorganic chemistry, 2nd ed., Instant notes series, Bios Scientific, London, ISBN 1-85996-289-0
- Cross RJ, Saunders M & Prinzbach H 1999, 'Putting Helium Inside Dodecahedrane', Organic Letters, vol. 1, no. 9, pp. 1479–1481, doi:10.1021/ol991037v
- Cverna F 2002, ASM ready reference: Thermal properties of metals, ASM International, Materials Park, Ohio, ISBN 0-87170-768-3
- Dalhouse University 2015, 'Dal chemist discovers new information about elemental boron', media release, 28 January, accessed 9 May 2015
- Deming HG 1952, General chemistry: An elementary survey, 6th ed., John Wiley & Sons, New York
- Desai PD, James HM & Ho CY 1984, Electrical resistivity of aluminum and manganese, Journal of Physical and Chemical Reference Data, vol. 13, no. 4, pp. 1131–1172, doi:10.1063/1.555725
- Donohoe J 1982, The Structures of the Elements, Robert E. Krieger, Malabar, Florida, ISBN 0-89874-230-7
- Douglade J & Mercier R 1982, 'Structure cristalline et covalence des liaisons dans le sulfate d'arsenic(III), As2(SO4)3', Acta Crystallographica Section B, vol. 38, no. 3, pp. 720–723, doi:10.1107/S056774088200394X
- Dunstan S 1968, Principles of chemistry, D. Van Nostrand Company, London
- Du Plessis M 2007, 'A Gravimetric Technique to Determine the Crystallite Size Distribution in High Porosity Nanoporous Silicon, in JA Martino, MA Pavanello & C Claeys (eds), Microelectronics Technology and Devices–SBMICRO 2007, vol. 9, no. 1, The Electrochemical Society, New Jersey, pp. 133–142, ISBN 978-1-56677-565-6
- Eby GS, Waugh CL, Welch HE & Buckingham BH 1943, The physical sciences, Ginn and Company, Boston, 1943
- Edwards PP & Sienko MJ 1983, 'On the occurrence of metallic character in the periodic table of the elements', Journal of Chemical Education, vol. 60, no. 9, pp. 691–696, doi:10.1021ed060p691
- Edwards PP 1999, 'Chemically engineering the metallic, insulating and superconducting state of matter' in KR Seddon & M Zaworotko (eds), Crystal engineering: The design and application of functional solids, Kluwer Academic, Dordrecht, pp. 409–431
- Edwards PP 2000, 'What, why and when is a metal?', in N Hall (ed.), The new chemistry, Cambridge University, Cambridge, pp. 85–114
- Edwards PP, Lodge MTJ, Hensel F & Redmer R 2010, '...a metal conducts and a non-metal doesn't', Philosophical Transactions of the Royal Society A: Mathematical, Physical and Engineering Sciences, vol. 368, pp. 941–965, doi:10.1098rsta.2009.0282
- Eichler R, Aksenov NV, Belozerov AV, Bozhikov GA, Chepigin VI, Dmitriev SN, Dressler R, Gäggeler HW, Gorshkov VA, Haenssler F, Itkis MG, Laube A, Lebedev VY, Malyshev ON, Oganessian YT, Petrushkin OV, Piguet D, Rasmussen P, Shishkin SV, Shutov, AV, Svirikhin AI, Tereshatov EE, Vostokin GK, Wegrzecki M & Yeremin AV 2007, 'Chemical characterization of element 112,' Nature, vol. 447, pp. 72–75, doi:10.1038/nature05761
- Endicott K 1998, 'The Trembling Edge of Science', Dartmouth Alumini Magazine, April, accessed 8 May 2015
- Emsley 1994, 'Science: Surprise legacy of Germany's Flying Bombs', New Scientist, no. 1910, January 29
- Emsley J 2001, Nature's building blocks: An A–Z guide to the elements, ISBN 0-19-850341-5
- Fraden JH 1951, 'Amorphous antimony. A lecture demonstration in allotropy', Journal of Chemical Education, vol. 28, no. 1, pp. 34–35, doi: 10.1021/ed028p34
- Furuseth S, Selte K, Hope H, Kjekshus A & Klewe B 1974, 'Iodine oxides. Part V. The crystal structure of (IO)2SO4', Acta Chemica Scandinavica A, vol. 28, pp. 71–76, doi:10.3891/acta.chem.scand.28a-0071
- Georgievskii VI 1982, 'Biochemical regions. Mineral composition of feeds', in VI Georgievskii, BN Annenkov & VT Samokhin (eds), Mineral nutrition of animals: Studies in the agricultural and food sciences, Butterworths, London, pp. 57–68, ISBN 0-408-10770-7
- Gillespie RJ & Robinson EA 1959, 'The sulphuric acid solvent system', in HJ Emeléus & AG Sharpe (eds), Advances in inorganic chemistry and radiochemistry, vol. 1, Academic Press, New York, pp. 386–424
- Glazov VM, Chizhevskaya SN & Glagoleva NN 1969, Liquid semiconductors, Plenum, New York
- Glinka N 1965, General chemistry, trans. D Sobolev, Gordon & Breach, New York
- Gösele U & Lehmann V 1994, 'Porous Silicon Quantum Sponge Structures: Formation Mechanism, Preparation Methods and Some Properties', in Feng ZC & Tsu R (eds), Porous Silicon, World Scientific, Singapore, pp. 17–40, ISBN 981-02-1634-3
- Greaves GN, Greer AL, Lakes RS & Rouxel T 2011, 'Poisson's ratio and modern materials', Nature Materials, vol. 10, pp. 823‒837, doi:10.1038/NMAT3134
- Greenwood NN & Earnshaw A 2002, Chemistry of the elements, 2nd ed., Butterworth-Heinemann, ISBN 0-7506-3365-4
- Gschneidner KA 1964, 'Physical properties and interrelationships of metallic and semimetallic elements,' Solid State Physics, vol. 16, pp. 275‒426, doi:10.1016/S0081-1947(08)60518-4
- Gupta A, Awana VPS, Samanta SB, Kishan H & Narlikar AV 2005, 'Disordered superconductors' in AV Narlikar (ed.), Frontiers in superconducting materials, Springer-Verlag, Berlin, p. 502, ISBN 3-540-24513-8
- Habashi F 2003, Metals from ores: an introduction to extractive metallurgy, Métallurgie Extractive Québec, Sainte Foy, Québec, ISBN 2-922686-04-3
- Manson SS & Halford GR 2006, Fatigue and Durability of Structural Materials, ASM International, Materials Park, OH, ISBN 0-87170-825-6
- Hem JD 1985, Study and interpretation of the chemical characteristics of natural water, paper 2254, 3rd ed., US Geological Society, Alexandria, Virginia
- Hampel CA & Hawley GG 1976, Glossary of chemical terms, Van Nostrand Reinhold, New York
- Hérold A 2006, 'An arrangement of the chemical elements in several classes inside the periodic table according to their common properties', Comptes Rendus Chimie, vol. 9, pp. 148–153, doi:10.1016/j.crci.2005.10.002
- Herzfeld K 1927, 'On atomic properties which make an element a metal', Phys. Rev., vol. 29, no. 5, pp. 701–705, doi:10.1103PhysRev.29.701
- Heslop RB & Robinson PL 1963, Inorganic chemistry: A guide to advanced study, Elsevier, Amsterdam
- Hill G & Holman J 2000, Chemistry in context, 5th ed., Nelson Thornes, Cheltenham, ISBN 0-17-448307-4
- Hiller LA & Herber RH 1960, Principles of chemistry, McGraw-Hill, New York
- Holtzclaw HF, Robinson WR & Odom JD 1991, General chemistry, 9th ed., DC Heath, Lexington, ISBN 0-669-24429-5
- Hopcroft MA, Nix WD & Kenny TW 2010, 'What is the Young's modulus of silicon?', Journal of Microelectromechanical Systems, vol. 19, no. 2, pp. 229‒238, doi:10.1109/JMEMS.2009.2039697
- Chemistry Views 2012, 'Horst Prinzbach (1931 – 2012)', Wiley-VCH, accessed 28 February 2015
- Hultgren HH 1966, 'Metalloids', in GL Clark & GG Hawley (eds), The encyclopedia of inorganic chemistry, 2nd ed., Reinhold Publishing, New York
- Hunt A 2000, The complete A-Z chemistry handbook, 2nd ed., Hodder & Stoughton, London
- Iler RK 1979, The chemistry of silica: solubility, polymerization, colloid and surface properties, and biochemistry, John Wiley, New York, ISBN 978-0-471-02404-0
- Jauncey GEM 1948, Modern physics: A second course in college physics, D. Von Nostrand, New York
- Jenkins GM & Kawamura K 1976, Polymeric carbons—carbon fibre, glass and char, Cambridge University Press, Cambridge
- Keenan CW, Kleinfelter DC & Wood JH 1980, General college chemistry, 6th ed., Harper & Row, San Francisco, ISBN 0-06-043615-8
- Keogh DW 2005, 'Actinides: Inorganic & coordination chemistry', in RB King (ed.), Encyclopedia of inorganic chemistry, 2nd ed., vol. 1, John Wiley & Sons, New York, pp. 2–32, ISBN 978-0-470-86078-6
- Klein CA & Cardinale GF 1992, 'Young's modulus and Poisson's ratio of CVD diamond', in A Feldman & S Holly, SPIE Proceedings, vol. 1759, Diamond Optics V, pp. 178‒192, doi:10.1117/12.130771
- Kneen WR, Rogers MJW & Simpson P 1972, Chemistry: Facts, patterns, and principles, Addison-Wesley, London
- Kovalev D, Timoshenko VY, Künzner N, Gross E & Koch F 2001, 'Strong Explosive Interaction of Hydrogenated Porous Silicon with Oxygen at Cryogenic Temperatures', Physical Review Letters, vol. 87, pp. 068301–1–06831-4, doi:10.1103/PhysRevLett.87.068301
- Kozyrev PT 1959, 'Deoxidized selenium and the dependence of its electrical conductivity on pressure. II', Physics of the solid state, translation of the journal Solid State Physics (Fizika tverdogo tela) of the Academy of Sciences of the USSR, vol. 1, pp. 102–110
- Kugler HK & Keller C (eds) 1985, Gmelin Handbook of Inorganic and Organometallic chemistry, 8th ed., 'At, Astatine', system no. 8a, Springer-Verlag, Berlin, ISBN 3-540-93516-9
- Lagrenaudie J 1953, 'Semiconductive properties of boron' (in French), Journal de chimie physique, vol. 50, nos. 11–12, Nov-Dec, pp. 629–633
- Lazaruk SK, Dolbik AV, Labunov VA & Borisenko VE 2007, 'Combustion and Explosion of Nanostructured Silicon in Microsystem Devices', Semiconductors, vol. 41, no. 9, pp. 1113–1116, doi:10.1134/S1063782607090175
- Legit D, Friák M & Šob M 2010, 'Phase Stability, Elasticity, and Theoretical Strength of Polonium from First Principles,' Physical Review B, vol. 81, pp. 214118–1–19, doi:10.1103/PhysRevB.81.214118
- Leith MM 1966, Velocity of sound in solid iodine, MSc thesis, University of British Coloumbia. Leith comments that, '... as iodine is anisotropic in many of its physical properties most attention was paid to two amorphous samples which were thought to give representative average values of the properties of iodine' (p. iii).
- Lide DR & Frederikse HPR (eds) 1998, CRC Handbook of chemistry and physics, 79th ed., CRC Press, Boca Raton, Florida, ISBN 0-849-30479-2
- Lidin RA 1996, Inorganic substances handbook, Begell House, New York, ISBN 1-56700-065-7
- Lindegaard AL and Dahle B 1966, 'Fracture phenomena in amorphous selenium', Journal of Applied Physics, vol. 37, no. 1, pp. 262‒66, doi:10.1063/1.1707823
- Mann JB, Meek TL & Allen LC 2000, 'Configuration energies of the main group elements', Journal of the American Chemical Society, vol. 122, no. 12, pp. 2780–2783, doi:10.1021ja992866e
- Marlowe MO 1970, Elastic properties of three grades of fine grained graphite to 2000°C, NASA CR‒66933, National Aeronautics and Space Administration, Scientific and Technical Information Facility, College Park, Maryland
- Martienssen W & Warlimont H (eds) 2005, Springer Handbook of Condensed Matter and Materials Data, Springer, Heidelberg, ISBN 3-540-30437-1
- Matula RA 1979, 'Electrical resistivity of copper, gold, palladium, and silver,' Journal of Physical and Chemical Reference Data, vol. 8, no. 4, pp. 1147–1298, doi:10.1063/1.555614
- McQuarrie DA & Rock PA 1987, General chemistry, 3rd ed., WH Freeman, New York
- Mendeléeff DI 1897, The Principles of Chemistry, vol. 2, 5th ed., trans. G Kamensky, AJ Greenaway (ed.), Longmans, Green & Co., London
- Mercier R & Douglade J 1982, 'Structure cristalline d'un oxysulfate d'arsenic(III) As2O(SO4)2 (ou As2O3.2SO3)', Acta Crystallographica Section B, vol. 38, no. 3, pp. 1731–1735, doi:10.1107/S0567740882007055
- Metcalfe HC, Williams JE & Castka JF 1966, Modern chemistry, 3rd ed., Holt, Rinehart and Winston, New York
- Mikulec FV, Kirtland JD & Sailor MJ 2002, 'Explosive Nanocrystalline Porous Silicon and Its Use in Atomic Emission Spectroscopy', Advanced Materials, vol. 14, no. 1, pp. 38–41, doi:10.1002/1521-4095(20020104)14:1<38::AID-ADMA38>3.0.CO;2-Z
- Moss TS 1952, Photoconductivity in the Elements, London, Butterworths
- Mott NF & Davis EA 2012, 'Electronic Processes in Non-Crystalline Materials', 2nd ed., Oxford University Press, Oxford, ISBN 978-0-19-964533-6
- Nakao Y 1992, 'Dissolution of Noble Metals in Halogen-Halide-Polar Organic Solvent Systems', Journal of the Chemical Society, Chemical Communications, no. 5, pp. 426–427, doi:10.1039/C39920000426
- Nemodruk AA & Karalova ZK 1969, Analytical chemistry of boron, R Kondor trans., Ann Arbor Humphrey Science, Ann Arbor, Michigan
- New Scientist 1975, 'Chemistry on the islands of stability', 11 Sep, p. 574, ISSN 1032-1233
- Noddack I 1934, 'On element 93', Angewandte Chemie, vol. 47, no. 37, pp. 653–655, doi:10.1002/ange.19340473707
- Olechna DJ & Knox RS 1965, 'Energy-band structure of selenium chains', Physical Review, vol. 140, pp. A986‒A993, doi:10.1103/PhysRev.140.A986
- Orton JW 2004, The story of semiconductors, Oxford University, Oxford, ISBN 0-19-853083-8
- Parish RV 1977, The metallic elements, Longman, London
- Partington JR 1944, A text-book of inorganic chemistry, 5th ed., Macmillan & Co., London
- Pauling L 1988, General chemistry, Dover Publications, NY, ISBN 0-486-65622-5
- Perkins D 1998, Mineralogy, Prentice Hall Books, Upper Saddle River, New Jersey, ISBN 0-02-394501-X
- Pottenger FM & Bowes EE 1976, Fundamentals of chemistry, Scott, Foresman and Co., Glenview, Illinois
- Qin J, Nishiyama N, Ohfuji H, Shinmei T, Lei L, Heb D & Irifune T 2012, 'Polycrystalline γ-boron: As hard as polycrystalline cubic boron nitride', Scripta Materialia, vol. 67, pp. 257‒260, doi:10.1016/j.scriptamat.2012.04.032
- Rao CNR & Ganguly P 1986, 'A new criterion for the metallicity of elements', Solid State Communications, vol. 57, no. 1, pp. 5–6, doi:10.1016/0038-1098(86)90659-9
- Rao KY 2002, Structural chemistry of glasses, Elsevier, Oxford, ISBN 0-08-043958-6
- Raub CJ & Griffith WP 1980, 'Osmium and sulphur', in Gmelin handbook of inorganic chemistry, 8th ed., 'Os, Osmium: Supplement,' K Swars (ed.), system no. 66, Springer-Verlag, Berlin, pp. 166–170, ISBN 3-540-93420-0
- Ravindran P, Fast L, Korzhavyi PA, Johansson B, Wills J & Eriksson O 1998, 'Density functional theory for calculation of elastic properties of orthorhombic crystals: Application to TiSi2', Journal of Applied Physics, vol. 84, no. 9, pp. 4891‒4904, doi:10.1063/1.368733
- Reynolds WN 1969, Physical properties of graphite, Elsevier, Amsterdam
- Rochow EG 1966, The metalloids, DC Heath and Company, Boston
- Rock PA & Gerhold GA 1974, Chemistry: Principles and applications, WB Saunders, Philadelphia
- Russell JB 1981, General chemistry, McGraw-Hill, Auckland
- Russell AM & Lee KL 2005, Structure-property relations in nonferrous metals, Wiley-Interscience, New York, ISBN 0-471-64952-X
- Sacks O 2001, Uncle Tungsten: Memories of a chemical boyhood, Alfred A Knopf, New York, ISBN 0-375-40448-1
- Sanderson RT 1960, Chemical periodicity, Reinhold Publishing, New York
- Sanderson RT 1967, Inorganic chemistry, Reinhold, New York
- Sanderson K 2012, 'Stinky rocks hide Earth's only haven for natural fluorine', Nature News, July, doi:10.1038/nature.2012.10992
- Schaefer JC 1968, 'Boron' in CA Hampel (ed.), The encyclopedia of the chemical elements, Reinhold, New York, pp. 73–81
- Sidgwick NV 1950, The chemical elements and their compounds, vol. 1, Clarendon, Oxford
- Sidorov TA 1960, 'The connection between structural oxides and their tendency to glass formation', Glass and Ceramics, vol. 17, no. 11, pp. 599–603, doi:10.1007BF00670116
- Sisler HH 1973, Electronic structure, properties, and the periodic law, Van Nostrand, New York
- Slezak 2014, 'Natural ball lightning probed for the first time', New Scientist, 16 January
- Slough W 1972, 'Discussion of session 2b: Crystal structure and bond mechanism of metallic compounds', in O Kubaschewski (ed.), Metallurgical chemistry, proceedings of a symposium held at Brunel University and the National Physical Laboratory on the 14, 15 and 16 July 1971, Her Majesty's Stationery Office [for the] National Physical Laboratory, London
- Slyh JA 1955, 'Graphite', in JF Hogerton & RC Grass (eds), Reactor handbook: Materials, US Atomic Energy Commission, McGraw Hill, New York, pp. 133‒154
- Smith A 1921, General chemistry for colleges, 2nd ed., Century, New York
- Sneed MC 1954, General college chemistry, Van Nostrand, New York
- Sommer AH, ‘Alloys of Gold with alkali metals’, Nature, vol. 152, p. 215, doi:10.1038/152215a0
- Soverna S 2004, 'Indication for a gaseous element 112,' in U Grundinger (ed.), GSI Scientific Report 2003, GSI Report 2004-1, p. 187, ISSN 0174-0814
- Stoker HS 2010, General, organic, and biological chemistry, 5th ed., Brooks/Cole, Cengage Learning, Belmont CA, ISBN 0-495-83146-8
- Stoye E 2014, 'Iridium forms compound in +9 oxidation state', Chemistry World, 23 October
- Sun H, Xu Z & Gao C 2013, 'Multifunctional, Ultra-Flyweight, Synergistically Assembled Carbon Aerogels', Advanced Materials,, vol. 25, no. 18, pp. 2554–2560, doi:10.1002/adma.201204576
- Sundara Rao RVG 1950, 'Elastic constants of orthorhombic sulphur,' Proceedings of the Indian Academy of Sciences - Section A, vol. 32, no. 4, pp. 275–278, doi:10.1007/BF03170831
- Sundara Rao RVG 1954, 'Erratum to: Elastic constants of orthorhombic sulphur', Proceedings of the Indian Academy of Sciences - Section A, vol. 40, no. 3, p. 151
- Swalin RA 1962, Thermodynamics of solids, John Wiley & Sons, New York
- Tilley RJD 2004, Understanding solids: The science of materials, 4th ed., John Wiley, New York
- Walker JD, Newman MC & Enache M 2013, Fundamental QSARs for metal ions, CRC Press, Boca Raton, ISBN 978-1-4200-8434-4
- White MA, Cerqueira AB, Whitman CA, Johnson MB & Ogitsu T 2015, 'Determination of Phase Stability of Elemental Boron', Angewandte Chemie International Edition, doi:10.1002/anie.201409169
- Wiberg N 2001, Inorganic chemistry, Academic Press, San Diego, ISBN 0-12-352651-5
- Wickleder MS, Pley M & Büchner O 2006, 'Sulfates of precious metals: Fascinating chemistry of potential materials', Zeitschrift für anorganische und allgemeine chemie, vol. 632, nos. 12–13, p. 2080, doi:10.1002/zaac.200670009
- Wickleder MS 2007, 'Chalcogen-oxygen chemistry', in FA Devillanova (ed.), Handbook of chalcogen chemistry: new perspectives in sulfur, selenium and tellurium, RSC, Cambridge, pp. 344–377, ISBN 978-0-85404-366-8
- Wilson JR 1965, 'The structure of liquid metals and alloys', Metallurgical reviews, vol. 10, p. 502
- Wilson AH 1966, Thermodynamics and statistical mechanics, Cambridge University, Cambridge
- Witczak Z, Goncharova VA & Witczak PP 2000, 'Irreversible effect of hydrostatic pressure on the elastic properties of polycrystalline tellurium', in MH Manghnani, WJ Nellis & MF Nicol (eds), Science and technology of high pressure: Proceedings of the International Conference on High Pressure Science and Technology (AIRAPT-17), Honolulu, Hawaii, 25‒30 July 1999, vol. 2, Universities Press, Hyderabad, pp. 822‒825, ISBN 81-7371-339-1
- Witt SF 1991, 'Dimethylmercury', Occupational Safety & Health Administration Hazard Information Bulletin, US Department of Labor, February 15, accessed 8 May 2015
- Wittenberg LJ 1972, 'Volume contraction during melting; emphasis on lanthanide and actinide metals', The Journal of Chemical Physics, vol. 56, no. 9, p. 4526, doi:10.1063/1.1677899
- Wulfsberg G 2000, Inorganic chemistry, University Science Books, Sausalito CA, ISBN 1-891389-01-7
- Young RV & Sessine S (eds) 2000, World of chemistry, Gale Group, Farmington Hills, Michigan
- Zhigal'skii GP & Jones BK 2003, Physical properties of thin metal films, Taylor & Francis, London, ISBN 0-415-28390-6
- Zuckerman & Hagen (eds) 1991, Inorganic reactions and methods, vol, 5: The formation of bonds to group VIB (O, S, Se, Te, Po) elements (part 1), VCH Publishers, Deerfield Beach, Fla, ISBN 0-89573-250-5
Periodic table (Large cells) | |||||||||||||||||||||||||||||||||
---|---|---|---|---|---|---|---|---|---|---|---|---|---|---|---|---|---|---|---|---|---|---|---|---|---|---|---|---|---|---|---|---|---|
1 | 2 | 3 | 4 | 5 | 6 | 7 | 8 | 9 | 10 | 11 | 12 | 13 | 14 | 15 | 16 | 17 | 18 | ||||||||||||||||
1 | H | He | |||||||||||||||||||||||||||||||
2 | Li | Be | B | C | N | O | F | Ne | |||||||||||||||||||||||||
3 | Na | Mg | Al | Si | P | S | Cl | Ar | |||||||||||||||||||||||||
4 | K | Ca | Sc | Ti | V | Cr | Mn | Fe | Co | Ni | Cu | Zn | Ga | Ge | As | Se | Br | Kr | |||||||||||||||
5 | Rb | Sr | Y | Zr | Nb | Mo | Tc | Ru | Rh | Pd | Ag | Cd | In | Sn | Sb | Te | I | Xe | |||||||||||||||
6 | Cs | Ba | La | Ce | Pr | Nd | Pm | Sm | Eu | Gd | Tb | Dy | Ho | Er | Tm | Yb | Lu | Hf | Ta | W | Re | Os | Ir | Pt | Au | Hg | Tl | Pb | Bi | Po | At | Rn | |
7 | Fr | Ra | Ac | Th | Pa | U | Np | Pu | Am | Cm | Bk | Cf | Es | Fm | Md | No | Lr | Rf | Db | Sg | Bh | Hs | Mt | Ds | Rg | Cn | Nh | Fl | Mc | Lv | Ts | Og | |
|